- 1Department of Nephrology, Leiden University Medical Center, Leiden, Netherlands
- 2Department of Clinical Epidemiology, Leiden University Medical Center, Leiden, Netherlands
- 3Department of Clinical Chemistry and Laboratory Medicine, Leiden University Medical Center, Leiden, Netherlands
- 4Department of Nephrology, Jeroen Bosch Hospital, Den Bosch, Netherlands
- 5Department of Nephrology and Hypertension, Antwerp University Hospital, Antwerp, Belgium
- 6Laboratory of Experimental Medicine and Paediatrics (LEMP), University of Antwerp, Antwerp, Belgium
Recipients of donation after circulatory death (DCD) kidneys are at high risk for delayed graft function (DGF) due to severe ischemia-reperfusion injury. We compared urinary biomarkers in predicting the duration of DGF with the tubular function slope (TFS) as the gold standard. In 89 DCD kidney transplant recipients, urinary TIMP-2, IGFBP7, B2M, NGAL, KIM1, CXCL9, and UMOD were quantified by LC-MS/MS analysis on postoperative days (PODs) 1, 4 and 10. Interstitial fibrosis and tubular atrophy (IF/TA) were assessed with protocol biopsies at POD 10. TFS was calculated with 99mTc-MAG3 renography. Predictive performance was compared with AUCs from ROC analyses. Of all 89 recipients, 22% experienced no (<7), 22% mild (≥7–14), 29% moderate (≥14-<21) and 26% severe (≥21 days) fDGF. The OR for the presence of IF/TA was 1.9 (95% CI:0.4; 10.0) for mild to moderate and 15.0 (95% CI:2.7; 84.8) for severe compared to no fDGF. At POD 4, urinary NGAL and fractional NGAL excretion (FE-NGAL) outperformed TFS and other biomarkers in predicting fDGF with AUCs of 0.97, 0.98 and 0.92, respectively. At POD10, FE-NGAL and PCR best predicted severe vs. mild to moderate fDGF, with AUCs of 0.74 and 0.76 versus 0.65 for TFS. Therefore, urinary NGAL and FE-NGAL may provide a viable alternative to 99mTcMAG3 renography for monitoring fDGF clearance or guiding kidney transplant biopsy to exclude additional acute rejection.
Introduction
For the majority of patients with end-stage kidney disease (ESKD), kidney transplantation is the preferred modality of renal replacement therapy (RRT), but with the ongoing gap between supply and demand, the waiting time while on dialysis is increasing [1]. Owing to the shortage of kidneys available for transplantation, many countries use donation after circulatory death (DCD) kidneys to expand the potential donor pool [2]. In the Netherlands, from 2017 to 2021, the relative contribution of DCD increased from 56% to 66% of all deceased kidney transplants [3].
Kidneys from DCD donors have a higher risk of severe ischemia-reperfusion injury (IRI) compared to kidneys from donation after brain death (DBD) or those from living donors. The longer initial warm ischemia time (WIT) to which DCD kidneys are exposed increases the risk of primary nonfunction (PNF) and delayed graft function (DGF), with the latter estimated to be up to 50% [1, 2]. DGF is a manifestation of ischemia-reperfusion or acute kidney injury (AKI), most commonly due to acute tubular necrosis (ATN), which causes post-transplantation oliguria or anuria, increased allograft immunogenicity and may increase the risk of early acute rejection [3–6].
Traditionally, DGF has been defined as the need for dialysis in the first week after kidney transplantation [7]. However, since the indication for dialysis is clinically determined by nephrologists on an individual basis, this dialysis-based definition is subjective and does not always reflect the lack of adequate glomerular filtration. Therefore, the function-based definition of DGF (fDGF) has been proposed as an alternative for retrospective evaluation. fDGF is established when serum creatinine fails to decrease by at least 10% per day for 3 consecutive days within the first week after kidney transplantation [8].
Predicting the duration of fDGF and monitoring for the occurrence of a concomitant early acute rejection episode remains a major challenge in the first weeks after kidney transplantation [9]. Sequential 99mTechnetium-mercaptoacetyltriglycine (99mTc-MAG3) renography can be used to identify the evolution of tubular function in the case of DGF. 99mTc-MAG3 renography allows for the calculation of a standardized tubular function slope (TFS), which reflects the 99mTc-MAG3 uptake by renal tubular cells during the first minutes after 99mTc-MAG3 injection [10, 11]. The TFS has previously been shown to be a sensitive biomarker of functioning proximal tubular epithelial cells (PTECs) and has been associated with fDGF and long-term graft function [10]. However, 99mTc-MAG3 renography is an expensive, invasive and time-consuming investigation.
99mTc-MAG3 is transported by organic anion transporters (OAT) expressed on the basolateral side of PTECs. Urinary biomarkers that identify tubular damage may offer a safer, quicker, and cheaper alternative to 99mTc-MAG3 renography. Several novel markers of urinary kidney injury, such as tissue inhibitor of metalloproteinases-2 (TIMP-2), insulin-like growth factor–binding protein 7 (IGFBP7), kidney injury molecule 1 (KIM-1), CXC Motif Chemokine Ligand 9 (CXCL9), uromodulin (UMOD), neutrophil gelatinase–associated lipocalin (NGAL) and beta-2 microglobulin (B2M) have been investigated. In particular, urinary markers of PTEC dysfunction may potentially be of interest to monitor the return of PTEC functionality. Both NGAL and B2M are freely filtered and almost completely reabsorbed via the luminal side of the PTECs (Figure 1). However, their exact pathophysiological role and diagnostic value in different etiologies of kidney injury remain unclear. Here, we investigated the relation between kidney transplant tissue quality and the duration of fDGF. Subsequently, we assessed the change in these novel urinary biomarkers of kidney injury in DCD kidney transplantation recipients stratified by fDGF duration as a measure of IRI severity. Finally, we compared the performance of these markers in predicting fDGF duration to that of TFS (chosen as the gold standard), in order to identify markers that can be used to easily monitor PTEC functionality.
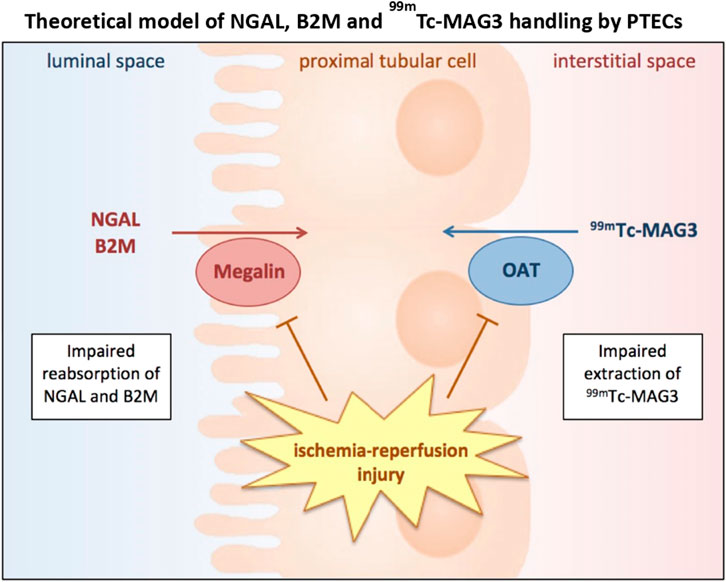
Figure 1. Ischemic and/or reperfusion injury of PTECs resulting in impaired 99mTc-MAG3 extraction via OATs on basolateral membranes as well as impaired NGAL and B2M reabsorption via megalin on apical membranes. Abbreviations: B2M, beta-2 microglobulin; NGAL, neutrophil gelatinase-associated lipocalin; PTEC, proximal tubular epithelial cell; OAT, organic anion transporter, 99mTc-MAG3, 99mTechnetium-mercaptoacetyltriglycine.
Materials and Methods
Study Design and Population
We included 89 out of 92 DCD kidney transplant recipients who participated in the Prospective Trial on Erythropoietin in Clinical Transplantation (PROTECT) [12]. Three PROTECT participants were excluded from the current project as they experienced PNF due to early graft thrombosis and urine samples were therefore not available. Briefly, PROTECT was a randomized, double-blind study comparing high-dose recombinant human erythropoietin (EPO)-β to placebo for the combined primary endpoint of primary nonfunction and DGF. All consecutive patients scheduled to receive a DCD kidney transplant at Leiden University Medical Center between August 2005 and December 2009 were approached to participate. High-dose EPO was administered to the recipient as an intravenous bolus on 3 consecutive days (total dose 100.000 IE) starting 3–4 h before the transplantation. All donors were controlled DCD cases (Maastricht Category III). Kidneys were allocated according to the allocation algorithm and matching criteria of both the standard Eurotransplant Kidney Allocation System (ETKAS; n = 73) and the Eurotransplant Senior Program (ESP; n = 16). At that time, Super Rapid (SR) procurement with cold preservation perfusion or Normothermic Regional Perfusion (NRP) technique was not yet available. There was no donor age limit for acceptance of DCD kidneys. The median age (46 years) of the DCD cases in the Netherlands was previously found to be significantly lower than that of heart-beating donors (48.5 years) in the period before the PROTECT study commenced [13]. All consecutive patients scheduled to receive a DCD kidney transplant were approached to participate in the PROTECT study. Exclusion criteria included panel-reactive antibodies (PRAs) > 60% at the time of transplantation, donor serum creatinine >150 μmol/L, first warm ischemic time (WIT) ≥ 45 min or cold ischemic time (CIT) > 24 h. The immunosuppressive regimen consisted of induction therapy with anti-CD25 antibody (daclizumab; intravenous bolus 100 mg preoperatively and on postoperative day (POD) 10) and triple maintenance therapy with mycophenolate mofetil, corticosteroids and delayed introduction of cyclosporine A (CsA) microemulsion. CsA (initial dose 3 mg/kg twice daily) was introduced on POD 4, with subsequent dosing according to the 12-hour area under the curve (AUC) targets of 5,400 ng/mL/h for the first 6 weeks after transplantation and 3,250 ng/mL/h thereafter. All patients received prophylactic therapy with trimethoprim/sulfamethoxazole 480 mg/day for 6 months against pneumocystis jiroveci pneumonia. The study was approved by the Ethical Committee of the Leiden University Medical Center (NCT00157300). At 1 year, there was no difference in the incidence or duration of DGF and/or primary nonfunction in patients treated with high-dose EPO versus placebo. Further details and results have been described previously [12].
Data Collection
Collected information included recipient age, sex, primary kidney disease, previous kidney transplantations, time on dialysis, donor age, sex, cause of death, hypertension, serum creatinine, and transplant characteristics including HLA mismatch, PRA, CIT and WIT. Additionally the kidney donor risk index (KDRI), a widely used tool to predict the risk of graft failure based on deceased donor characteristics, was calculated [14].
Definition of Functional DGF (fDGF)
DGF was defined according to the functional definition (fDGF); a failure of serum creatinine to decrease spontaneously by ≥ 10% daily on three successive days during the week after transplantation or dialysis requirement [8]. The second of three consecutive days was used as the index day to group patients by duration of fDGF, resulting in 4 groups of <7 days, ≥7–14 days, ≥14–21 days and ≥21 days. fDGF ≥7 or ≥21 days can be considered as having either fDGF or severe fDGF, respectively.
Laboratory Measures
At the time of the study, urine and serum samples were collected at PODs 1-7, POD 10, at 6 weeks and 6 months after transplantation. For the current project, we only used urine data on PODs 1, 4 and 10, at 6 weeks and 6 months after transplantation since TFS and serum analyses for creatinine, B2M and NGAL were performed only on those days. Urine osmolality was determined by freezing point depression using an Osmo-Station (Auto & Stat model OM-6060, Arkray Inc., Kyoto, Japan). Urinary total protein (TP) was determined by turbidimetry (Cat. No. 05171954190), B2M by immunoturbidimetry (Cat. No. 08047430190), and creatinine by an enzymatic method (Cat. No. 3263991190), all using a Cobas C8000 c702 (Roche Diagnostics, Mannheim, Germany).
Urinary NGAL, IGFBP7, KIM-1, TIMP2, CXCL9, UMOD, SLC22A2 and nephrin were quantified in 36 batches between January 2021 and November 2021, using an in-house developed multiplex liquid chromatography tandem mass spectrometry (LC-MS/MS) test. The preanalytical and analytical phases of this LC-MS/MS test followed the standard operating procedure described elsewhere [15, 16]. To ensure LC-MS/MS performance, a system suitability test was carried out prior to each analysis batch of study samples (a maximum of 81 samples per batch). To monitor LC-MS/MS performance over time, two urine-based internal quality control (IQC) samples were prepared and analyzed with the study samples. The IQC results were monitored in Levey-Jennings charts and the test performance was considered stable over 1 year [17, 18]. All urine samples were stored for 10–15 years and underwent one to two freeze-thaw cycles. However, it is important to note that LC-MS/MS tests are relatively insensitive to freezing and thawing of samples.
With the serum and urinary biomarker and creatinine values, fractional excretion (FE) of B2M and NGAL were calculated, analogous to the FE of sodium. In analogy to the protein-to-creatinine ratio (PCR), we calculated the ratios of B2M/(TIMP2) and NGAL/(TIMP2). Theoretically, TIMP2 or IGFBP7 could substitute creatinine as a glomerular filtration marker, whereas B2M and NGAL are actively reabsorbed in proximal epithelial cells.
Protocol Kidney Biopsy
Per the protocol, all recipients underwent a kidney transplant biopsy on POD 10. Adequate biopsy samples were available for 64 recipients. Biopsies were unavailable (n = 25) due to: withdrawal of consent (n = 7), insufficient tissue (n = 11) or staining issues (n = 7). An experienced pathologist scored all biopsies according to the semi-quantitative ATN score and assessed interstitial fibrosis and tubular atrophy (IF/TA) according to the Banff 2009 classification, as a proxy for donor-derived fibrosis [19, 20]. Biopsy results have been published previously [21].
99mTc-MAG3 Renography
99mTc-MAG3 renography was performed on PODs 1, 4 and 10 to calculate the tubular function slope (TFS) [10]. Briefly, a bolus of 100 MBq of 99mTc-MAG3 was injected and frames were recorded with a large-field-of-view gamma camera (Toshiba GCA501S), at 1-second intervals for 120 frames, then at 20-second intervals for 90 frames. The 99mTc-MAG3 dose was corrected for extravasation. TFS was calculated by analyzing radiopharmaceutical uptake by renal tubular cells using a nuclear medicine computer (MAPS 10000 Web Link Medical). Two regions of interest were drawn semi-automatically; one around the graft and one representing the background. Subsequently, a background-subtracted graft and dose-adjusted 99mTc-MAG3 curve were generated. During the first two minutes of the renography two phases can be recognized in the graft: a rapidly ascending phase, representing the perfusion of the kidney, followed by a second phase of tubular extraction. Using a linear fit (least-squares error estimate), the slope of the second phase of this curve was determined and defined as TFS.
Statistical Analysis
First, baseline recipient, donor and transplant characteristics are presented here as mean (±SD) or number (proportion) for all recipients and stratified by fDGF duration. Second, we studied the relation between baseline KDRI, fDGF duration and IF/TA presence in the kidney biopsy on POD 10, using logistic regression analysis to investigate the clinical relevance of severe fDGF. Third, we studied the relation between fDGF duration and endogenous creatinine clearance (ECC) at 6 weeks and 12 months, using logistic regression analysis for the outcome ECC ≥40 vs. < 40 mL/min, and linear regression analysis for the change in ECC in mL/min.
Fourth, we calculated the median (interquartile range [IQR]) values of TFS, urine volume, creatinine, PCR, and creatinine-corrected NGAL, B2M, TIMP2, IGFBP7, KIM-1, CXCL9, UMOD, and FE-NGAL and FE-B2M on PODs 1, 4 and 10, for all recipients and stratified by fDGF duration.
Fifth, we calculated Pearson correlation coefficients to study the association between TFS and urinary creatinine, PCR, standardized creatinine-corrected NGAL, B2M and TIMP2, IGFBP7, KIM-1, CXCL9, UMOD, and FE-NGAL and FE-B2M, at PODs 1, 4 and 10. A p-value < 0.05 was considered statistically significant.
Sixth, we calculated AUCs with receiver operating characteristic (ROC) analyses for fDGF presence at PODs 1 and 4, and fDGF severity at POD 10 as predicted by standardized TFS, urinary creatinine, PCR and standardized creatinine-corrected urinary NGAL, B2M, TIMP2, IGFBP7, KIM-1, CXCL9, UMOD, FE-NGAL and FE-B2M. In all regression and ROC analyses, markers were divided by their SD to normalize their distributions.
Missing urine samples and measurements are reported in Supplementary Table S3. At PODs 4 and 10, 32 DCD kidney transplant recipients had complete data for ROC analyses. We conducted a complete case analysis to compare results with our main analyses. All analyses were performed using R version 4.0.3 (R Core Team, Vienna, Austria).
Results
Cohort Characteristics
Urine and serum samples were available for 89 DCD recipients. The mean age of the recipients was 54 (±13) years and 62 (70%) were men. For 86 (96%) recipients, this was their first kidney transplant, with a mean dialysis vintage of 4.4 (±2.5) years. Only one recipient (1%) received a preemptive transplant. Of the 89 recipients, 20 (22%) had no fDGF (<7 days), 20 (22%) had mild fDGF (≥7–14 days), 26 (29%) had moderate fDGF (≥14 to <21 days), and 23 (26%) had severe fDGF (≥21 days). The mean age of donors was 46 (±15) years and 55% were men. Donors for recipients with fDGF (≥7 days) were older, more often men and had higher KDRI scores than those without fDGF. At POD 10, IF/TA was more often present, TFS and ECC were lower in those with fDGF, especially severe fDGF, compared to those without fDGF. Further donor, recipient and transplant characteristics are summarized in Table 1. Detailed causes of primary kidney disease are shown in Supplementary Table S1.
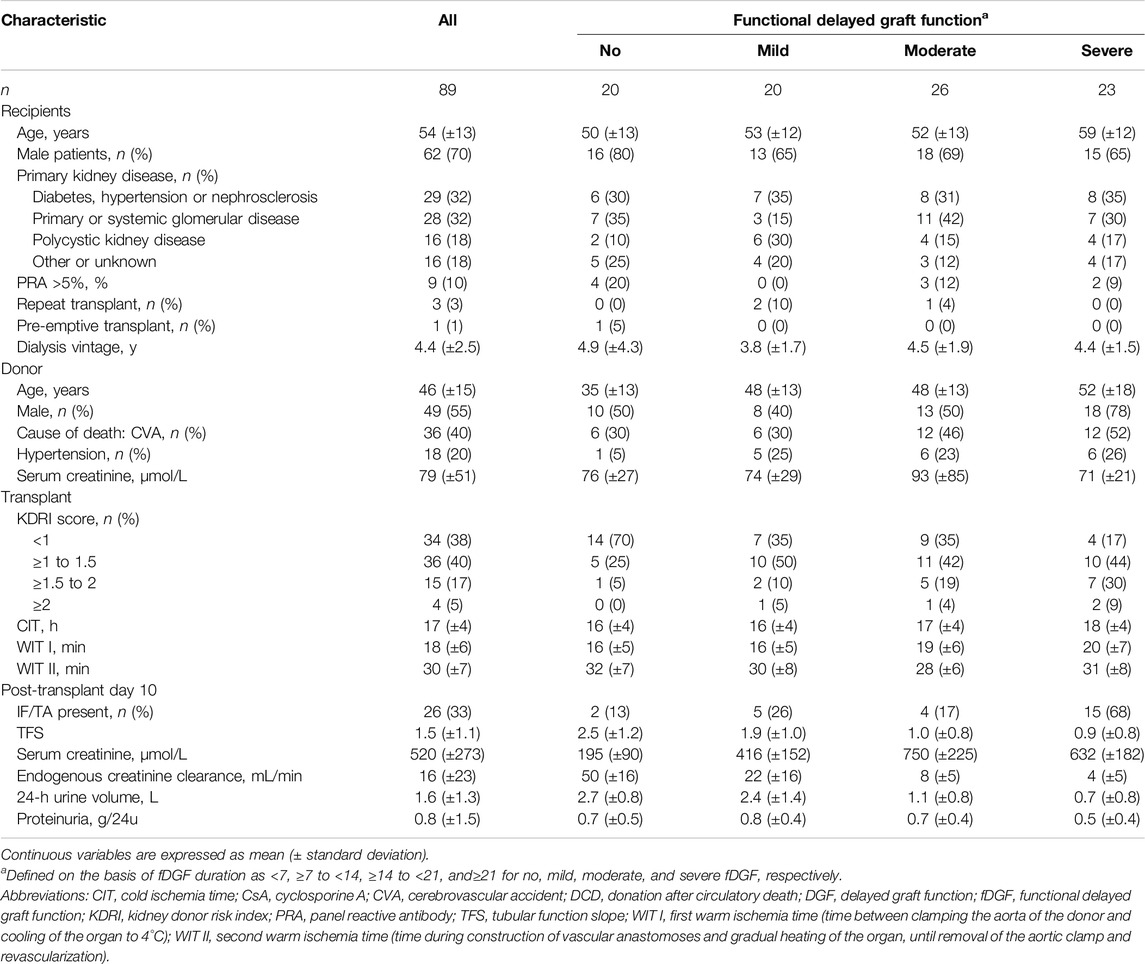
Table 1. Recipient, donor, and transplant characteristics of 89 donation after circulatory death kidney transplantations.
fDGF Duration: KDRI, Donor-Derived Fibrosis and 1-Year Renal Function
Using a KDRI of <1.0 as reference, scores of ≥1.0 to 1.5 and ≥1.5 were associated with an OR (95% CI) for POD 10 kidney biopsy IF/TA presence of 8.3 (1.7; 40.9) and 15.6 (2.8; 86.8), respectively. Compared to no fDGF, fDGF was associated with an OR of 4.2 (0.9; 20.1) for IF/TA presence at POD 10. For mild and moderate compared to no fDGF, the OR for IF/TA presence was 1.9 (0.4; 10.0), whereas this was 15.0 (2.7; 84.8) for severe fDGF (Table 2).
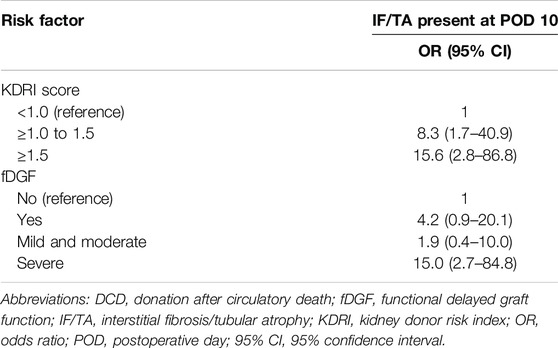
Table 2. Risk of IF/TA according to KDRI or fDGF scores compared to the reference category in 89 DCD recipients.
Longer duration of fDGF was clearly associated with a lower eGFR or ECC. The OR (95% CI) for ECC <40 vs. ≥ 40 mL/min at 6 weeks and 12 months for mild, moderate fDGF or severe fDGF compared to no fDGF were 5.2 (1.4; 19.3), 2.0 (0.5; 8.1) and 113.3 (10.8; 1,192.8), and 4.9 (0.6; 40.4), 3.5 (0.4; 30.5), and 9.5 (1.0; 89.0), respectively. For mild, moderate or severe compared to no fDGF, the RR (95% CI) for decrease in ECC in mL/min was −13.7 (−22.8; −4.7), −6.2 (−14.1; 1.8) at 6 weeks, and −30.4 (−39.6; −21.1), and −12.0 (−23.9; −0.1), −7.1 (−19.1; 4.9), and −24.2 (−38.7;−9.7) at 12 months, respectively (Table 3).
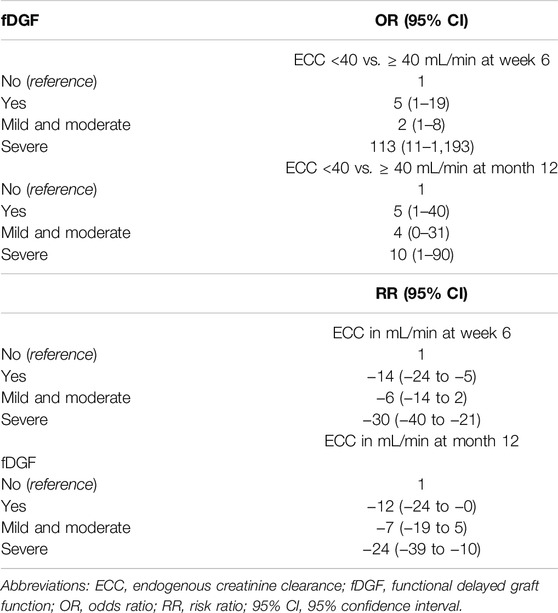
Table 3. Among 89 recipients, the odds and risk ratios (95% CI) for the relation between duration of fDGF and endogenous creatinine clearance at 6 weeks and 12 months, calculated using logistic and linear regression analysis.
Kidney Injury Markers, Conventional Markers and TFS Over Time
Figure 2 shows median (IQR) levels at PODs 1, 4 and 10 of TFS, conventional markers (urinary creatinine and PCR), and the urinary creatinine-standardized kidney injury markers B2M and NGAL across all recipients and stratified by fDGF duration. Detailed levels of these markers along with urine volume, TIMP2, IGFBP7, KIM1, CXCL9, UMOD and FE-B2M and FE-NGAL are shown in Supplementary Table S2 and Supplementary Figure S1. Pearson’s correlation coefficients between standardized TFS and creatinine-corrected urinary markers at PODs 1, 4 and 10 are shown in Supplementary Table S3 and Supplementary Figure S2. Correlations with TFS were generally poor or modest (as expected), with the strongest at POD 10 for urinary B2M and FE-B2M with coefficients of −0.53 (p = 0.00) and −0.56 (p = 0.00), respectively.
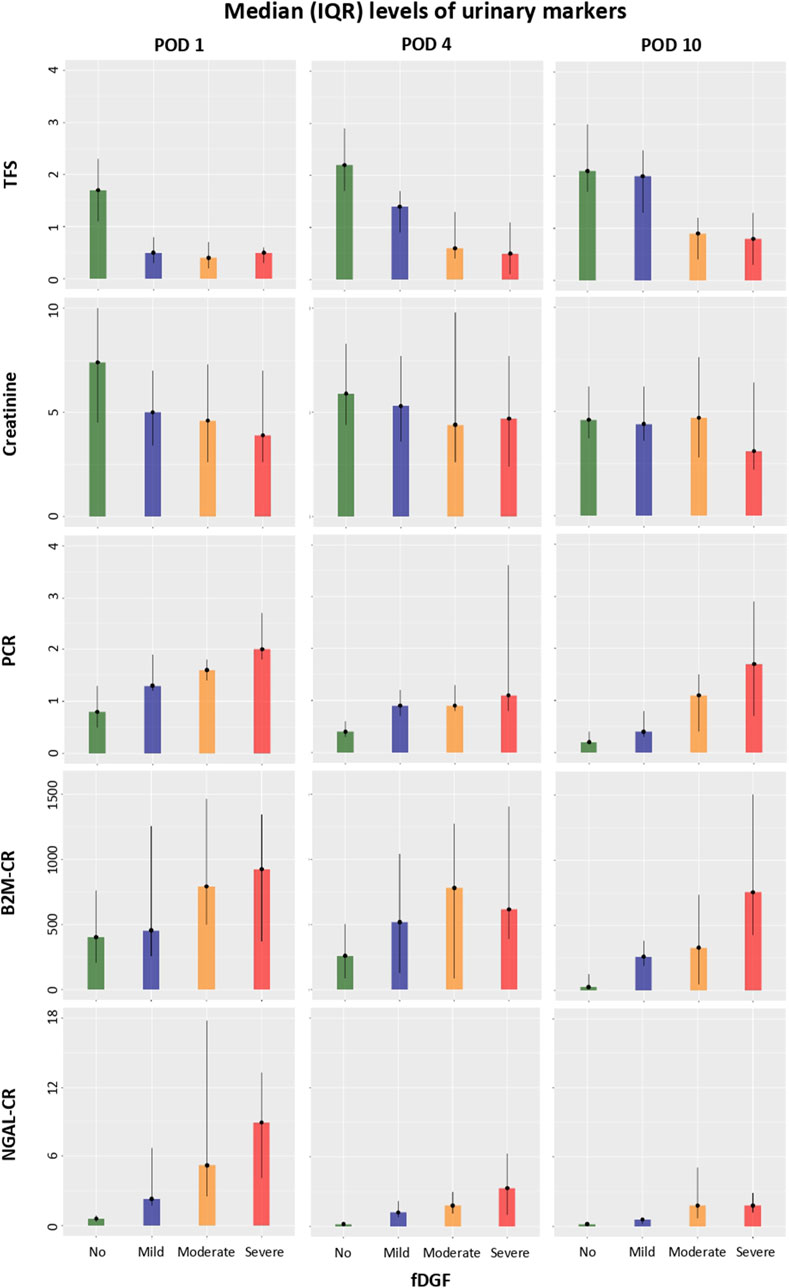
Figure 2. Among 89 recipients, and stratified by fDGF duration at PODs 1, 4 and 10 after DCD kidney transplantation, the median (IQR) levels of TFS and creatinine-corrected urinary markers. *Defined based on fDGF duration as <7, ≥7 to <14, ≥14 to <21, ≥21 for no, mild, moderate and severe fDGF, respectively. Abbreviations: B2M, beta-2 microglobulin; CR, creatinine ratio; DCD, donation after circulatory death; fDGF, functional delayed graft function; NGAL, neutrophil gelatinase-associated lipocalin; PCR, protein to creatinine ratio; POD, postoperative day; TFS, tubular function slope; u, urinary.
Kidney Injury Markers Compared to TFS for Prediction of fDGF
At POD 1, AUCs (95% CI) for predicting the presence of fDGF (yes vs. no) were 0.90 (0.81; 0.99), 0.73 (0.60; 0.86), 0.77 (0.62; 0.92), 0.68 (0.53; 0.82), 0.55 (0.40; 0.71), 0.89 (0.76; 1.00), and 0.81 (0.68; 0.93), for TFS, creatinine, PCR, B2M, FE-B2M, NGAL and FE-NGAL, respectively. At POD 4, NGAL and FE-NGAL outperformed TFS with AUCs of 0.97 (0.90; 1.00) and 0.98 (0.93; 1.00), respectively, compared to 0.92 (0.86; 0.98) for TFS (Figure 3; Table 4; Supplementary Figure S3). At POD 10, FE-NGAL, PCR and NGAL/TIMP2 performed best for severe vs. mild to moderate fDGF prediction, as AUCs were 0.74 (0.55; 0.93), 0.76 (0.58; 0.94) and 0.72 (0.53; 0.91), respectively, compared to 0.65 (0.48; 0.82) for TFS (Table 4; Supplementary Figure S3). A complete case analysis including 32 DCD kidney transplant recipients yielded similar results (Supplementary Tables S4, S5).
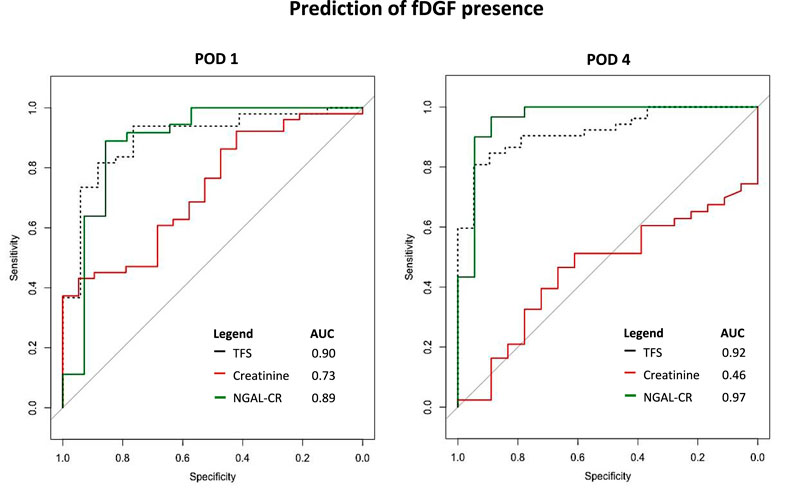
Figure 3. ROC analysis for the prediction of fDGF presence (yes vs. no) by standardized TFS and standardized creatinine-corrected urinary NGAL at PODs 1 and 4 after DCD kidney transplantation. Abbreviations: AUC, area under the curve; CR, creatinine ratio; DCD, donation after circulatory death; fDGF, functional delayed graft function; NGAL, neutrophil gelatinase-associated lipocalin; POD, postoperative day; TFS, tubular function slope.
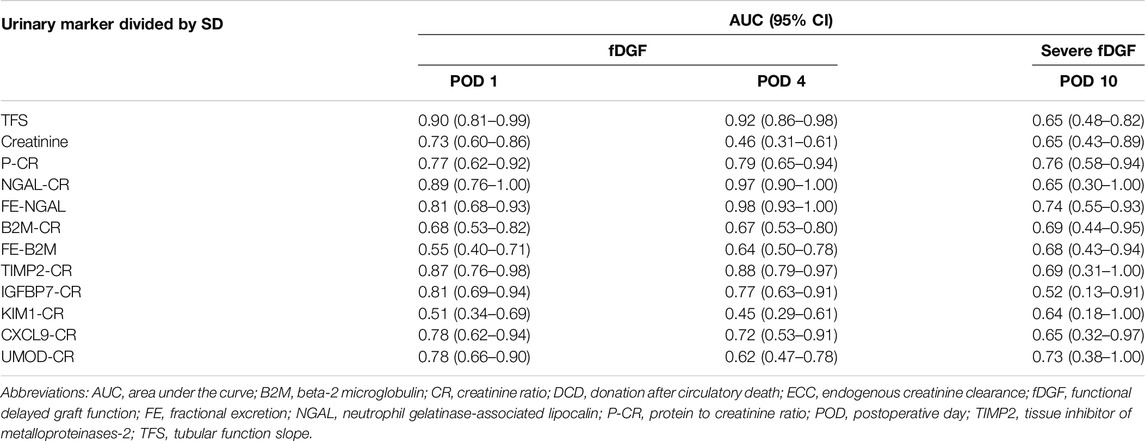
Table 4. Area under the curve (95% CI) from ROC analysis for the prediction of fDGF presence at PODs 1 and 4, and fDGF severity at POD 10 by standardized TFS and standardized creatinine-corrected urinary markers at PODs 1, 4 and 10 after DCD kidney transplantation.
Discussion
NGAL and FE-NGAL, measured at PODs 1, 4 and 10 outperformed TFS in predicting fDGF presence and severity in 89 DCD kidney transplantation recipients. fDGF severity was strongly related to IF/TA presence in POD 10 kidney biopsies and lower kidney function at 6 weeks and 12 months after transplantation. Daily monitoring of urinary NGAL or FE-NGAL in the first days after kidney transplantation may provide an alternative to sequential 99mTcMAG3 renography to follow PTEC function recovery and fDGF resolution.
DGF is associated with acute rejection and has an adverse impact on longer-term kidney function and patient outcomes [8, 22, 23]. Indeed, in our study the severity of fDGF was strongly associated with the presence of (donor-derived) IF/TA in the kidney biopsies, observed in 68% of those with fDGF ≥21 days compared to only 33% for the entire cohort. Distinguishing early acute rejection from a primarily insufficient renal mass remains challenging in the first weeks after kidney transplantation [9]. This is especially true in DCD kidney recipients in whom severe IRI is higher than in those receiving kidneys from living or DBD donors [1, 2]. We used the TFS as the gold standard to assess the severity of kidney injury. The TFS quantifies the tubular extraction rate of 99mTc-MAG3 through the OAT, providing insight into the overall quality and functional recovery of PTECs. Although proven accurate and effective in identifying DGF, 99mTc-MAG3 renography remains invasive, time-consuming and costly, rendering sequential TFS less appealing for routine clinical use. Our results indicate that, among both conventional and novel urinary biomarkers, FE-NGAL in particular has the potential to replace TFS allowing daily monitoring of IRI resolution.
The urinary biomarkers TIMP-2, IGFBP7, KIM-1, CXCL9, UMOD, NGAL and B2M may reflect different aspects of renal pathophysiology, although research is ongoing. For example, TIMP-2 and IGFBP7 are thought to act as markers of cellular stress and G1 cell cycle arrest, aiding in the early detection of AKI [24–26]. KIM-1 is a marker of kidney injury, primarily expressed in damaged proximal tubular cells [24, 27, 28]. CXCL9 is associated with the immune response and renal inflammation. UMOD, on the other hand, is the most abundant protein in normal urine and plays a role in kidney function and urinary tract maintenance [24].
Urinary B2M and NGAL have been well-researched as markers of proximal tubular dysfunction. B2M binds to major histocompatibility complex I (MHC-I)/human leukocyte antigen I (HLA-I) on nucleated cells [29]. NGAL is synthesized by epithelial tissues, including distal tubular epithelial cells [29]. In normal kidneys, B2M and NGAL undergo unhindered glomerular filtration and are almost entirely reabsorbed by PTECs [27, 29, 30]. After any surgical procedure that causes damage to the epithelial tissue, both plasma B2M and NGAL will increase. Following PTEC injury, reabsorption of B2M and NGAL is impaired, increasing urinary excretion. Since B2M and NGAL are normally reabsorbed via the apical PTEC membrane, and 99mTc-MAG3 (used to determine the TFS) at the basolateral membrane, we hypothesized that these markers specifically would be equally accurate in the prediction of fDGF. However, since B2M (as compared to NGAL) is more abundantly present in tissues, blood levels of B2M will increase more than NGAL following surgery. The subsequent high fractional urinary excretion of B2M, independent of PTEC function, disrupts the interpretability of urinary B2M as a marker of PTEC injury. Indeed, we found that compared to TFS, NGAL-CR or FE-NGAL were stronger predictors than B2M-CR for the presence of fDGF at POD 1 and POD 4, and severe fDGF at POD 10. Thus, NGAL-CR and FE-NGAL may provide an alternative to 99mTcMAG3 renography for following fDGF resolution and anticipating poorer long-term kidney function.
Sequential measurements of NGAL-CR or FE-NGAL in the first days following kidney transplantation can be used to monitor the recovery of PTEC function. Through this, transplant recipients prone to experience severe fDGF may be identified early on, as NGAL will not yet be reabsorbed due to PTEC dysfunction. A decrease in urinary NGAL will indicate restoration of PTEC functionality (Figure 4). In our DCD transplant recipient population, (FE-)NGAL levels (corrected for urinary creatinine and the population standard deviation) on separate days already predicted the presence and severity of fDGF when compared to the TFS as the gold standard. Sequential monitoring of NGAL will be more informative, especially considering the fluctuating nature and wide interpatient variation of NGAL. Of course, future research in larger populations is needed to determine reference levels and to interpret when a relative increase or decrease in NGAL would be clinically relevant. Furthermore, NGAL levels should always be interpreted in the context of other clinical characteristics such as diuretic volume. Decreasing urinary NGAL levels, indicating recovery of PTEC function, could guide the indication for or timing of kidney transplant biopsy to exclude the occurrence of another acute rejection episode and subsequent treatment. Therefore, in the future, NGAL testing may also improve long-term outcomes.
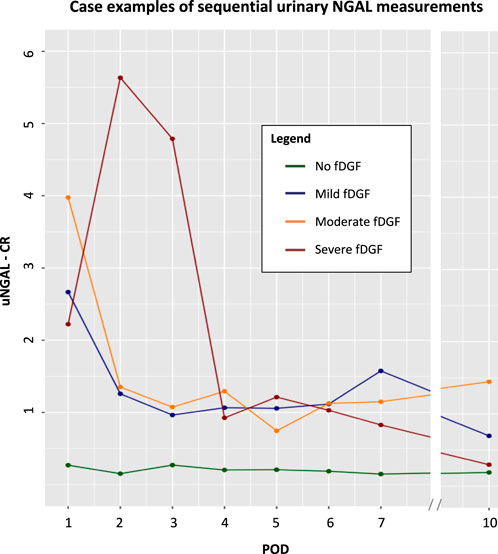
Figure 4. Case examples of urinary (creatinine-corrected) NGAL measurements among 4 representative DCD kidney transplantation recipients with no, mild, moderate or severe fDGF*. *Defined based on fDGF duration as <7, ≥7 to <14, ≥14 to <21, ≥21 days for no, mild, moderate and severe fDGF, respectively. Abbreviations: CR, creatinine ratio; DCD, donation after circulatory death; fDGF, functional delayed graft function; NGAL, neutrophil gelatinase-associated lipocalin; POD, postoperative day; u, urinary.
The current body of evidence is, however, insufficient to support large-scale implementation of routine NGAL measurement. Furthermore, sequential NGAL measurement could also be beneficial for other indications other than DGF resolution monitoring, such as early AKI monitoring, for instance during treatment with potentially nephrotoxic chemotherapeutics. If sequential NGAL testing is validated in future research as reliable for monitoring DGF resolution or timely diagnosis of early AKI, it could guide timely intervention and thereby significantly reduce costs by preventing severe complications [31, 32]. Finally, with the development of easier analytical techniques, such as our in-house developed multiplex liquid chromatography tandem mass spectrometry (LC-MS/MS) test, biomarker analysis costs will be reduced [15, 16].
There are several strengths to our study. First, we are comparing urinary markers to the TFS (99mTcMAG3 renography) shortly after DCD kidney transplantation for the first time. Since recipients of DCD kidneys are at higher risk for severe IRI, adequate monitoring of kidney injury is especially important in this group. Second, by using an in-house developed multiplex LC-MS/MS test, we were able to efficiently and reliably assess urinary levels of nine injury markers simultaneously. Third, by comparing previously proposed markers of kidney injury in recipients of DCD kidneys at high risk of severe IRI, we aimed to perform a hypothesis-generating study that may focus future research efforts. Fourth, there was no selection of participants in the PROTECT study since all consecutive patients scheduled to receive a DCD kidney transplant were approached to participate. This included expanded criteria donors (ECD) and kidneys allocated via the Eurotransplant Senior Program (ESP). Fifth, organ procurement techniques have significantly improved since the time of the PROTECT study (2005–2009). Nowadays, procurement techniques such as SR and NRP reduce the severity of IRI in DCD kidney transplantation. Consequently, with more heterogeneity in IRI severity among current DCD kidney recipients, the application of these biomarkers (after external validation) for early fDGF prediction may become especially relevant. Our results should ideally be validated in representative cohorts that include these different options in procurement and allocation strategies.
Nevertheless, our study has some limitations. First, this was a single-center study, which may limit the generalizability of our results to other centers or countries. However, our experience with DCD kidney transplantation allowed us to use this relatively large cohort to investigate the added value and patterns of these biomarkers in DCD kidney recipients. Second, we found a high percentage of IF/TA in the kidney transplant biopsies at POD 10. This is to be expected considering that these were DCD kidney transplant recipients who were transplanted between August 2005 and December 2009. The consecutive DCD kidneys offered included expanded criteria donors (ECD) and kidneys allocated via the Eurotransplant Senior Program (ESP). The presence of IF/TA was strongly related to the KDRI score, which is largely driven by donor age. Hence, IF/TA presence will mainly be donor-derived. The high percentage of IF/TA in these DCD kidney transplants will have affected the levels of urinary biomarkers. Hence, these results should not be generalized to cohorts other than DCD kidney transplant recipients. Furthermore, as transplantation techniques have improved since 2009, our results should ideally be validated in representative cohorts that include these different options in procurement and allocation strategies. Third, no data exist on whether the high-dose EPO administered as an intervention to a part of the study population may have affected the validity of our biomarker analyses. However, as there was no difference in the incidence or duration of DGF and/or primary nonfunction in patients treated with high-dose EPO versus placebo, we do not expect EPO administration to have affected our biomarker results. Fourth, due to the limited sample size and missing data, this study may have been underpowered to some extent, particularly to detect associations between urinary biomarker levels and concurrent kidney function and kidney function decline. This will, however, not have been inferred from our descriptive analysis assessing the patterns of these urinary biomarkers and their correlation with TFS. Third, part of the missing urine samples will be due to anuria. However, since anuria itself indicates DGF, it will be less relevant to measure biomarker levels in anuric patients. Finally, all urine samples included in our analysis were stored for 10–15 years and underwent one to two freeze-thaw cycles. However, it is important to note that LC-MS/MS tests are relatively insensitive to freezing and thawing of samples.
In conclusion, we found that NGAL and FE-NGAL, measured on PODs 1, 4 or 10 after DCD kidney transplantation in 89 recipients, performed better than the TFS in predicting the presence and severity of fDGF. fDGF severity was strongly related to the KDRI, the presence of donor-derived fibrosis in day-10 protocol kidney biopsies, and resulted in inferior kidney graft function 12 months after kidney transplantation. Daily urinary NGAL and FE-NGAL monitoring in the first days after kidney transplantation may provide an alternative to sequential 99mTcMAG3 renography to follow PTEC function recovery and fDGF resolution, and may guide the timing of a kidney biopsy to exclude the occurrence of an additional acute rejection episode.
Data Availability Statement
The datasets presented in this article are not readily available because as our data could be used to identify individuals, privacy concerns prevent us from allowing them to be publically available. Nonetheless, we are open to make our data available for collaboration conditional on agreement on privacy matters and appropriate usage of the data. For this, please contact the corresponding author. Requests to access the datasets should be directed to ER, ZS5uLm0ucm9vaWpAbHVtYy5ubA==.
Ethics Statement
The studies involving humans were approved by Ethical Committee of the Leiden University Medical Center (NCT00157300). The studies were conducted in accordance with the local legislation and institutional requirements. The participants provided their written informed consent to participate in this study.
Author Contributions
ER conceptualized the study design, performed the data analysis and participated in the writing of the paper; TD developed the in-house LC-MS/MS test, conducted the urinary biomarker analyses and reviewed the paper; EH conceptualized the study design, supervised the data analysis and participated in the writing and review of the paper; FR conducted the serum and urinary analyses and reviewed the paper; FD reviewed the paper; CK participated in the original PROTECT study data collection and reviewed the paper; CC supervised the urinary biomarker analyses and reviewed the paper; JF participated in the original PROTECT study data collection, conceptualized the study design and reviewed the paper. All authors contributed to the article and approved the submitted version.
Funding
The author(s) declare that no financial support was received for the research and/or publication of this article.
Conflict of Interest
The authors declare that the research was conducted in the absence of any commercial or financial relationships that could be construed as a potential conflict of interest.
Supplementary Material
The Supplementary Material for this article can be found online at: https://www.frontierspartnerships.org/articles/10.3389/ti.2025.13818/full#supplementary-material
References
1. Hoogland, ER, Snoeijs, MG, Winkens, B, Christaans, MHL, and van Heurn, LWE. Kidney Transplantation from Donors after Cardiac Death: Uncontrolled versus Controlled Donation. Am J Transpl (2011) 11(7):1427–34. doi:10.1111/j.1600-6143.2011.03562.x
2. Mannon, RB. Delayed Graft Function: The AKI of Kidney Transplantation. Nephron (2018) 140(2):94–8. doi:10.1159/000491558
3. Lechevallier, E, Dussol, B, Luccioni, A, Thirion, X, Vacher-Copomat, H, Jaber, K, et al. Posttransplantation Acute Tubular Necrosis: Risk Factors and Implications for Graft Survival. Am J Kidney Dis (1998) 32:984–91. doi:10.1016/s0272-6386(98)70073-3
4. Gueler, F, Shushakova, N, Mengel, M, Hueper, K, Chen, R, Liu, X, et al. A Novel Therapy to Attenuate Acute Kidney Injury and Ischemic Allograft Damage after Allogenic Kidney Transplantation in Mice. PLoS One (2015) 10(1):e0115709. doi:10.1371/journal.pone.0115709
5. Zhao, H, Alam, A, Soo, AP, George, AJT, and Ma, D. Ischemia-Reperfusion Injury Reduces Long Term Renal Graft Survival: Mechanism and beyond. EBioMedicine (2018) 28:31–42. doi:10.1016/j.ebiom.2018.01.025
6. Siedlecki, A, Irish, W, and Brennan, DC. Delayed Graft Function in the Kidney Transplant. Am J Transpl (2011) 11(11):2279–96. doi:10.1111/j.1600-6143.2011.03754.x
7. Perico, N, Cattaneo, D, Sayegh, MH, and Remuzzi, G. Delayed Graft Function in Kidney Transplantation. Lancet (2004) 364(9447):1814–27. doi:10.1016/S0140-6736(04)17406-0
8. Boom, H, Mallat, MJ, de Fijter, JW, Zwinderman, AH, and Paul, LC. Delayed Graft Function Influences Renal Function, but Not Survival. Kidney Int (2000) 58(2):859–66. doi:10.1046/j.1523-1755.2000.00235.x
9. Justiz Vaillant, AA, Misra, S, and Fitzgerald, BM. Acute Transplantation Rejection. In: StatPearls [Internet]. Treasure Island (FL): StatPearls Publishing (2024).
10. El-Maghraby, TA, Boom, H, Camps, JA, Blokland, KAK, Zwinderman, AH, Paul, LC, et al. Delayed Graft Function Is Characterized by Reduced Functional Mass Measured by (99m)Technetium-Mercaptoacetyltriglycine Renography. Transplantation (2002) 74(2):203–8. doi:10.1097/00007890-200207270-00010
11. Benjamens, S, Pol, RA, de Geus-Oei, LF, de Vries, APJ, Glaudemans, AWJM, Berger, SP, et al. Can Transplant Renal Scintigraphy Predict the Duration of Delayed Graft Function? A Dual Center Retrospective Study. PLoS One (2018) 13(3):e0193791. doi:10.1371/journal.pone.0193791
12. Aydin, Z, Mallat, MJ, Schaapherder, AF, van Zonneveld, AJ, van Kooten, C, Rabelink, TJ, et al. Randomized Trial of Short-Course High-Dose Erythropoietin in Donation after Cardiac Death Kidney Transplant Recipients. Am J Transpl (2012) 12(7):1793–800. doi:10.1111/j.1600-6143.2012.04019.x
13. Keizer, KM, de Fijter, JW, Haase-Kromwijk, BJ, and Weimar, W. Non-Heart-Beating Donor Kidneys in the Netherlands: Allocation and Outcome of Transplantation. Transplantation (2005) 79(9):1195–9. doi:10.1097/01.tp.0000160765.66962.0b
14. Rao, PS, Schaubel, DE, Guidinger, MK, Andreoni, KA, Wolfe, RA, Merion, RM, et al. A Comprehensive Risk Quantification Score for Deceased Donor Kidneys: The Kidney Donor Risk Index. Transplantation (2009) 88(2):231–6. doi:10.1097/TP.0b013e3181ac620b
15. van Duijl, TT, Ruhaak, LR, Smit, NPM, Pieterse, MM, Romijn, FPHTM, Dolezal, N, et al. Development and Provisional Validation of a Multiplex LC-MRM-MS Test for Timely Kidney Injury Detection in Urine. J Proteome Res (2021) 20(12):5304–14. doi:10.1021/acs.jproteome.1c00532
16. van Duijl, TT, de Rooij, ENM, Treep, MM, Koelemaij, ME, Romijn, FPHTM, Hoogeveen, EK, et al. Urinary Kidney Injury Biomarkers Are Associated with Ischemia-Reperfusion Injury Severity in Kidney Allograft Recipients. Clin Chem (2023) 69(8):924–35. doi:10.1093/clinchem/hvad086
17. van Duijl, TT, Ruhaak, LR, van Kooten, C, de Fijter, JW, and Cobbaert, CM. Multiplex LC-MS/MS Testing for Early Detection of Kidney Injury: A Next-Generation Alternative to Conventional Immunoassays? J Appl Lab Med (2022) 7(4):923–30. doi:10.1093/jalm/jfac024
18. van Duijl, TT, Ruhaak, LR, Hoogeveen, EK, de Mutsert, R, Rosendaal, FR, le Cessie, S, et al. Reference Intervals of Urinary Kidney Injury Biomarkers for Middle-Aged Men and Women Determined by Quantitative Protein Mass Spectrometry. Ann Clin Biochem (2022) 59(6):420–32. doi:10.1177/00045632221121780
19. Goujon, JM, Hauet, T, Menet, E, Levillain, P, Babin, P, and Carretier, M. Histological Evaluation of Proximal Tubule Cell Injury in Isolated Perfused Pig Kidneys Exposed to Cold Ischemia. J Surg Res (1999) 82:228–33. doi:10.1006/jsre.1998.5526
20. Sis, B, Mengel, M, Haas, M, Colvin, RB, Halloran, PF, Racusen, LC, et al. Banff '09 Meeting Report: Antibody Mediated Graft Deterioration and Implementation of Banff Working Groups. Am J Transpl (2010) 10:464–71. doi:10.1111/j.1600-6143.2009.02987.x
21. Bank, JR, van der Pol, P, Vreeken, D, Monge-Chaubo, C, Bajema, IM, Schlagwein, N, et al. Kidney Injury Molecule-1 Staining in Renal Allograft Biopsies 10 Days after Transplantation Is Inversely Correlated with Functioning Proximal Tubular Epithelial Cells. Nephrol Dial Transpl (2017) 32(12):2132–41. doi:10.1093/ndt/gfx286
22. Yarlagadda, SG, Coca, SG, Formica, RN, Poggio, ED, and Parikh, CR. Association between Delayed Graft Function and Allograft and Patient Survival: A Systematic Review and Meta-Analysis. Nephrol Dial Transpl (2009) 24(3):1039–47. doi:10.1093/ndt/gfn667
23. Gill, J, Dong, J, Rose, C, and Gill, JS. The Risk of Allograft Failure and the Survival Benefit of Kidney Transplantation Are Complicated by Delayed Graft Function. Kidney Int (2016) 89(6):1331–6. doi:10.1016/j.kint.2016.01.028
24. Kashani, K, Al-Khafaji, A, Ardiles, T, Artigas, A, Bagshaw, SM, Bell, M, et al. Discovery and Validation of Cell Cycle Arrest Biomarkers in Human Acute Kidney Injury. Crit Care (2013) 17:R25. doi:10.1186/cc12503
25. Bihorac, A, Chawla, LS, Shaw, AD, Al-Khafaji, A, Davison, DL, Demuth, GE, et al. Validation of Cell-Cycle Arrest Biomarkers for Acute Kidney Injury Using Clinical Adjudication. Am J Respir Crit Care Med (2014) 189:932–9. doi:10.1164/rccm.201401-0077OC
26. Hoste, EAJ, McCullough, PA, Kashani, K, Chawla, LS, Joannidis, M, Shaw, AD, et al. Derivation and Validation of Cutoffs for Clinical Use of Cell Cycle Arrest Biomarkers. Nephrol Dial Transpl (2014) 29:2054–61. doi:10.1093/ndt/gfu292
27. Ostermann, M, Philips, BJ, and Forni, LG. Clinical Review: Biomarkers of Acute Kidney Injury: Where Are We Now? Crit Care (2012) 16(5):233. doi:10.1186/cc11380
28. Ichimura, T, Hung, CC, Yang, SA, Stevens, JL, and Bonventre, JV. Kidney Injury Molecule-1: A Tissue and Urinary Biomarker for Nephrotoxicant-Induced Renal Injury. Am J Physiol Ren Physiol (2004) 286(3):F552–63. doi:10.1152/ajprenal.00285.2002
29. Schrezenmeier, EV, Barasch, J, Budde, K, Westhoff, T, and Schmidt-Ott, KM. Biomarkers in Acute Kidney Injury -Pathophysiological Basis and Clinical Performance. Acta Physiol (2017) 219(3):554–72. doi:10.1111/apha.12764
30. Argyropoulos, CP, Chen, SS, Ng, YH, Roumelioti, ME, Shaffi, K, Singh, PP, et al. Rediscovering Beta-2 Microglobulin as a Biomarker across the Spectrum of Kidney Diseases. Front Med (2017) 4:73. doi:10.3389/fmed.2017.00073
31. Jacobsen, E, Sawhney, S, Brazzelli, M, Aucott, L, Scotland, G, Aceves-Martins, M, et al. Cost-Effectiveness and Value of Information Analysis of NephroCheck and NGAL Tests Compared to Standard Care for the Diagnosis of Acute Kidney Injury. BMC Nephrol (2021) 22(1):399. doi:10.1186/s12882-021-02610-9
Keywords: DCD donation, NGAL, urinary biomarkers, delayed graft function (DGF), kidney transplantation
Citation: de Rooij ENM, van Duijl TT, Hoogeveen EK, Romijn FPHTM, Dekker FW, van Kooten C, Cobbaert CM and de Fijter JW (2025) Urinary NGAL Outperforms 99mTc-MAG3 Renography in Predicting DCD Kidney Graft Function. Transpl. Int. 38:13818. doi: 10.3389/ti.2025.13818
Received: 18 September 2024; Accepted: 15 April 2025;
Published: 12 May 2025.
Copyright © 2025 de Rooij, van Duijl, Hoogeveen, Romijn, Dekker, van Kooten, Cobbaert and de Fijter. This is an open-access article distributed under the terms of the Creative Commons Attribution License (CC BY). The use, distribution or reproduction in other forums is permitted, provided the original author(s) and the copyright owner(s) are credited and that the original publication in this journal is cited, in accordance with accepted academic practice. No use, distribution or reproduction is permitted which does not comply with these terms.
*Correspondence: Esther N. M. de Rooij, ZS5uLm0ucm9vaWpAbHVtYy5ubA==
†ORCID: Esther N. M. de Rooij, orcid.org/0000-0003-3017-2885; Tirsa T. van Duijl, orcid.org/0000-0001-8069-6631; Ellen K. Hoogeveen, orcid.org/0000-0002-5482-2013; Fred P.H.T.M. Romijn, orcid.org/0000-0003-4470-7959; Friedo W. Dekker, orcid.org/0000-0002-2433-2494; Cees van Kooten, orcid.org/0000-0002-6257-0899; Christa M. Cobbaert, orcid.org/0000-0003-3565-1404; Johan W. de Fijter, orcid.org/0000-0003-3076-5584