- 1Department of Kidney Disease and Transplant Immunology, Aichi Medical University School of Medicine, Nagakute, Japan
- 2Biostatistics Center, Kurume University, Kurume, Japan
- 3Department of Transplant Surgery, Japanese Red Cross Aichi Medical Center Nagoya Daini Hospital, Nagoya, Japan
- 4Department of Transplant Surgery, Toyohashi Municipal Hospital, Toyohashi, Japan
- 5Department of Nephrology, Masuko Memorial Hospital, Nagoya, Japan
- 6Department of Renal Transplant Surgery, Aichi Medical University School of Medicine, Nagakute, Japan
- 7Department of Nephrology, Nagoya University Graduate School of Medicine, Nagoya, Japan
In ABO blood group incompatible kidney transplantation (ABO-I), potential issues on acute antibody-mediated rejection (ABMR) remain to be solved. This study aimed to assess the risk factors of acute ABMR using recipient- or donor-derived specimens. Quantitative analysis of A/B antigen expression was conducted in 104 donor kidney tissues (Kt), platelets (Plt), and red blood cells (RBC) by immunohistochemical staining or flow cytometry (FCM). ABO-I pre-transplant recipient serum samples (ABMR = 12, non-ABMR = 27) were extracted by propensity score matching. Anti-A antibody titers of IgM, IgG and IgG subclasses, and C1q binding ability (%) on antibody were measured using RBC-FCM. No association was observed between ABMR and A/B antigen expression levels in donor’s Plt, RBC, or Kt. In recipient’s sample, C1q-IgG binding ability was significantly higher in the ABMR group than in the non-ABMR group (C1q−IgG: 9.04% vs. 5.93% p = 0.049). Neither the A/B antigen expression level in donors (grafts) nor anti-blood group IgG/IgM antibodies in recipient sera before desensitization seemed to influence ABMR incidence in ABO-I. In contrast, C1q-IgG binding ability could be a potential predictor for ABMR in ABO-I.
Introduction
Effective desensitization therapy has improved the outcomes of ABO-incompatible (ABO-I) kidney transplantation [1, 2]. However, the graft survival rate of ABO-I is slightly inferior to ABO-Identical/compatible kidney transplantation (ABO-Id/C) [3, 4]. This may be due not to acute antibody-mediated rejection (ABMR) but adverse effects such as infectious diseases [5]. The recent SARS-COV-2 pandemic has caused fear of infection in immunosuppressed transplant patients [6, 7]. Furthermore, patients under rituximab (RIT) treatment showed low vaccine efficacy [8]. Therefore, unnecessary desensitization therapy should be avoided. Optimization of immunosuppressive therapy (IST) including desensitization by risk stratification of acute ABMR (i.e., reduction of desensitization regimen for patients with a low risk) may further improve outcomes in ABO-I.
The intensity of the antigen-antibody reaction is defined by the density of antigen expression and the amount of antibody. Determining the antigen expression in vascular endothelial cells (EC) of donor grafts before transplantation could provide important information on donor risk factors. However, since the kidney tissues (Kt) of donors are not commonly available before transplantation, platelets (Plt) and red blood cells (RBC) in the peripheral blood, and which express blood group A/B antigens [9–12], were examined to test whether their expression levels correlate with the amount of A/B antigen in the graft’s EC. Although the carbohydrate binding protein [13] or carbohydrate chain of a glycan precursor [14, 15] properties of A/B antigens seem to differ between RBC and EC, it would be important to know whether the A/B antigen expression levels in RBC or Plt can reflect those in EC. Currently, measuring the anti-A/B antibody titer in the recipient serum by hemagglutination is widely used as a main pre-test in ABO-I, but anti-A/B antibody titer alone may be insufficient in clinical settings [16, 17].
Another risk factor, this time in recipients, is related to a difference in complement activation ability between IgG subclasses: both IgG1 and IgG3 have higher ability than IgG2 or IgG4 [18, 19]. Furthermore, the IgG1, IgG2, IgG3, and IgG4 distribution in peripheral blood differs from person to person [14, 20]. However, whether the patterns of IgG subclasses in the recipient’s pre-transplant blood can be a risk factor of acute ABMR in ABO-I remains unknown. In contrast, in HLA (Human Leukocyte antigen) -incompatible kidney transplantation (HLA-I), there are reports on the value of IgG subclass post-transplant measurement in recipients as a prognostic marker [21–23]. C1q, the first component of the complement activation through the classical pathway, binding ability to donor specific HLA antibody (DSA) has been associated with ABMR and graft loss [24, 25], whereas the correlation between ABMR incidence in ABO-I and C1q binding ability to anti-A/B antibody has not been reported yet.
In this study, we examined whether A/B antigen expression in the donor (Kt, RBC, and Plt) and C1q binding ability against donor RBC, and anti-A antibodies in recipient sera could predict ABMR in ABO-I.
Materials and Methods
Study Design and Patients
[Donor Patients] Case Control Study 1
Kidney grafts from 104 living donors (A group: n = 54, B group: n = 32, AB group: n = 18) were transplanted at the Japanese Red Cross Aichi Medical Center Nagoya Daini Hospital, between 1998 and 2017. Among 72 patients expressing A antigen in the grafts, 39 patients and 33 patients were ABO-I and ABO-Id/C, respectively. Five of the 39 ABO-I had ABMR. Similarly, only one in 30 ABO-I expressing blood group B in the graft showed acute ABMR (Figure 1A).
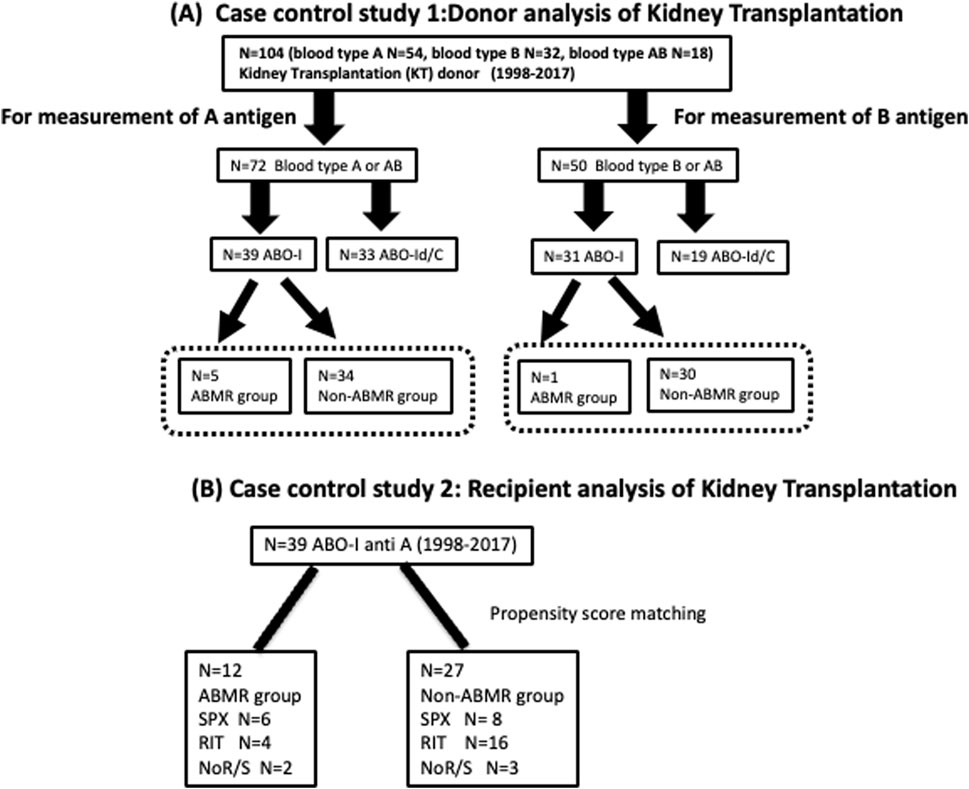
Figure 1. Flow chart of patient selection (A) Case control study 1: Donor analysis of kidney transplantation Plt, RBC, and Kt were collected from 104 ABO-I and ABO-Id/C donors to measure A/B antigen expression. Then, in ABO-I, we compared whether there was a difference in A antigen expression between the ABMR and non-ABMR groups. Plt, platelets; RBC, red blood cells; Kt, kidney tissues; ABO-I, ABO-incompatible kidney transplantation; ABO-Id/C, ABO-identical or compatible kidney transplantation. (B) Case control study 2: Recipient analysis of kidney transplantation. The background of anti-A 39 patients who underwent ABO-I at the Japanese Red Cross Aichi Medical Center Nagoya Daini Hospital between 1998 and 2017 were compared between ABMR and non-ABMR groups. RIT, rituximab; SPX, splenectomy; NoR/S, neither rituximab nor splenectomy.
[Recipient Patients] Case Control Study 2
The backgrounds of 42 patients with blood group A mismatch who underwent ABO-I at the Japanese Red Cross Aichi Medical Center Nagoya Daini Hospital between 1998 and 2017 were compared after classification into the ABMR and non-ABMR groups (Figure 1B). Patients in the non-ABMR group were extracted based on propensity score matching; there was no significant difference in age, sex, blood group, desensitization therapy, and maintenance IST of recipient and donor patients. Patient characteristics are summarized in Table 1. The study was performed in accordance with the guidelines of the Declaration of Helsinki, after approval from the hospital’s institutional ethical committee of Aichi Medical University School of Medicine (authorization number 15-092, 15-H072).
Desensitization Protocol
ABO-I recipients were pretreated with mycophenolate mofetil (MMF) from day −14, double-filtration plasmapheresis (DFPP) and either splenectomy (SPX), rituximab (RIT) (200 mg/body; twice; days −14 and −1, available from 2008), or neither (due to low anti-A/B antibody titers). Preoperative DFPP was routinely performed four times (days −6, −4, −2, and −1) in RIT or SPX and twice (days −2 and −1) in NoR/S.
Immunosuppression Protocol
All transplant recipients received 500 mg methylprednisolone intravenously before graft reperfusion and 20 mg of basiliximab intravenously on days 0 and 4. The immunosuppressive regimen consisted of a calcineurin inhibitor (cyclosporine or tacrolimus), an antimetabolite (MMF or mizoribine) or mammalian target of rapamycin inhibitor (everolimus, available from 2008), and prednisolone. The dosage of all oral immunosuppressive medications, except prednisolone, was strictly adjusted according to pharmacokinetics (AUC 0–4 h or trough level). Cyclophosphamide was used as an antimetabolite only in case of SPX.
ABMR Diagnosis
In this study, recipients with preformed DSA were not extracted. Whenever rejection was clinically suspected, an episodic biopsy was performed. The diagnosis of rejection was made by a pathologist at the Japanese Red Cross Aichi Medical Center Nagoya Daini Hospital. If no anti-donor HLA Abs were detected at the time of rejection, the diagnosis of ABMR due to anti-A or anti-B Abs was made using the pathology findings of ABMR (Banff 1997, 2001, 2005, 2007, 2013, 2017) during the study period 1998-2017.
Immunohistochemical Staining of Kt
Donor renal tissue of 1-h biopsy after transplantation was formalin-fixed and embedded in paraffin. Staining for blood group A and B antigen was performed on 1 µm thick paraffin embedded sections. After deparaffinization, sections were incubated with a monoclonal mouse IgM anti-A antibody (clone MH04,3D3; Ortho Clinical Diagnostics, Tokyo, Japan) and a monoclonal mouse IgM anti-B antibody (clone NE11.19,5A5,3D4; Ortho Clinical Diagnostics) as primary antibodies. Next, sections were incubated with Dako Envision detection System (DAKO, Glostrup, Denmark) as second antibody. Peroxidase activity was visualized by staining with a 3,3′-Diaminobenzidine, tetrahydrochloride (DAB) solution. Immunostained slides were scanned in a virtual slide microscopy (VS120, Olympus, Tokyo, Japan). In this study, the DAB stain area of A/B antigens, was measured using the image analysis software Tissuemorph DP (Visiopharm, Hoersholm, Denmark). A/B antigen expression was analyzed in three selected renal glomeruli; Tissuemorph DP shows the area of DAB stain in green, the nuclei in blue, and a region of interest (ROI) around blue dotted line. The index of A/B antigen immunopositivity was the ratio of the total DAB stain area and total ROI area (Max DAB/ROI value; Supplementary Figure S1) [26].
Flow Cytometry Analysis of Blood Type A, B Antigen Expression on Plt and RBC
Platelet-rich plasma (PRP) was prepared by centrifugation of anti-coagulated whole blood in acid-citrate-dextrose (ACD) tube at 250 g for 15 min. Then, the PRP was diluted three times with 20% ACD in Plt buffer (0.14 M NaCl, 5 mM KCl, 1 mM MgSO4, and 10 mM HEPES, pH 7.4), and centrifuged at 750 g for 2.5 min to form platelet pellets. Plt were stabilized by fixation in paraformaldehyde at a final concentration of 1%. RBC was collected by centrifugation at 1,000 g from citric acid-treated blood and washed twice with PBS (−) containing 0.2% bovine serum albumin and 0.1% NaN3 (wash buffer). Then, they were incubated with 3 mg/mL dimethyl suberimidate dihydrochroride (DMS) in 0.1 M Na2CO3 containing 0.15 M NaCl and 0.1 mM EDTA at 37°C for 20 min to prevent agglutination. DMS-treated RBC were washed with wash buffer twice and suspended in wash buffer at 1% concentration. For the detection of blood group A/B antigen in Plt and RBC using flow cytometry (FACSCanto II, Becton Dickinson, San Jose, CA, United States), 4.0 ×106 Plt and 4.5 × 105 RBC were incubated with monoclonal mouse IgM anti-A or B antibody (Ortho Clinical Diagnostic) for 20 min at room temperature. Fluorescein (FITC)-labeled goat anti-mouse IgM (American Qualex Antibodies, San Clemente, CA) was used as secondary antibody. A/B antigen expression levels were analyzed by the mean fluorescence intensity (MFI).
Detection of Anti-A IgG, IgM, and IgG Antibody Titers in Patient Serum
For the detection of anti-A antibody titer in patient pre-treatment serum using RBC flow cytometry, 30 µL of 1 × 107/mL DMS-treated RBCs and 15 µL of heat-inactivated patient serum were incubated in 96-well plates for 20 min at room temperature. After three washes with 0.1% BSA in PBS (−), RBC were incubated with a diluted secondary antibody, either FITC-labeled rabbit anti-human IgG, IgM (DAKO) or R-phycoerythrin (R-PE)-labeled mouse IgG1, IgG2, IgG3, IgG4 (SouthernBiotech, Birmingham, AL, United States). The stained RBC were analyzed using high-throughput flow cytometry (FACS Canto II High Throughput Sampler option, Becton Dickinson), which allows simultaneous testing of large patient’s samples in 96-well plates. The anti-A antibody isohemagglutinin antibody titers for IgG and IgM were serially measured as previously reported [27].
Detection of Complement C1q (C1q−IgG and C1q−IgG+IgM) Binding Ability in Patient Serum
To degrade IgM antibodies, heat-inactivated patient serum was incubated with 5 mM dithiothreitol (DTT) at 37°C for 30 min. At first 30 µL of 1 × 107/mL DMS-treated RBC and 15 µL of patient serum (DTT treated or non-treated) were incubated for 20 min at room temperature. After three washes with 0.1% BSA in PBS (−), RBC were incubated with 5 µL of complement component C1q from human serum (Sigma-Aldrich, St Louis, MO, United States) in PBS (−) at room temperature for 20 min. Then, after adding 50 µL of ×20 diluted FITC-labeled rabbit polyclonal anti-human C1q antibody (ab4223; Abcam plc, Cambridge, United Kingdom), RBC were incubated at room temperature for 20 min. After washing RBC twice with 0.1% BSA in PBS (−), RBC were measured using flow cytometry (FACS Canto II, Becton Dickinson). To assess C1q binding ability, RBC reacted with C1q; secondary antibody were used only as negative controls and threshold lines were drawn at 3% C1q binding ability of the AB blood type serum and compared in terms of positivity rate (%).
Statistical Analysis
The variability of groups with different units was expressed by the coefficient of variation (CV). The Mann–Whitney U test was used to compare two groups of continuous variables. Medians with a 25th and 75th percentile were calcurated. The cut-off value was determined by receiver operator characteristic curve (ROC) analysis using Youden index. Moreover, Fisher’s exact test in a 2 × 2 contingency table was used to compare categorical data between groups. P values < 0.05 were considered statistically significant. Statistical analyses were performed using GraphPad Prism ver 5.03 and JMP ver 13.2.
Results
Individual Differences in Blood Type A/B Antigen Expression in Donors’ Plt, RBC, and Kt
We measured A/B antigen expression levels in the Plt, RBC, and Kt of 104 donors (Figure 1A), A antigen: n = 72 [ABMR(+) n = 5, ABMR(−) n = 34, ABO-Id/C n = 33], B antigen: n = 50 [ABMR(+) n = 1, ABMR(−) n = 30, ABO-Id/C n = 19] of donor patients (Figure 2). The inter-individual differences in both A and B antigen in Plt were larger than those in RBC and Kt [CV; 0.74 (Plt) vs. 0.19 (RBC) and 0.26 (Kt) in A antigen, 2.04 (Plt) vs. 0.23 (RBC) and 0.44 (Kt) in B antigen]. No correlation in A/B antigen expression levels was observed between Plt, RBC, and Kt (Figure 2).
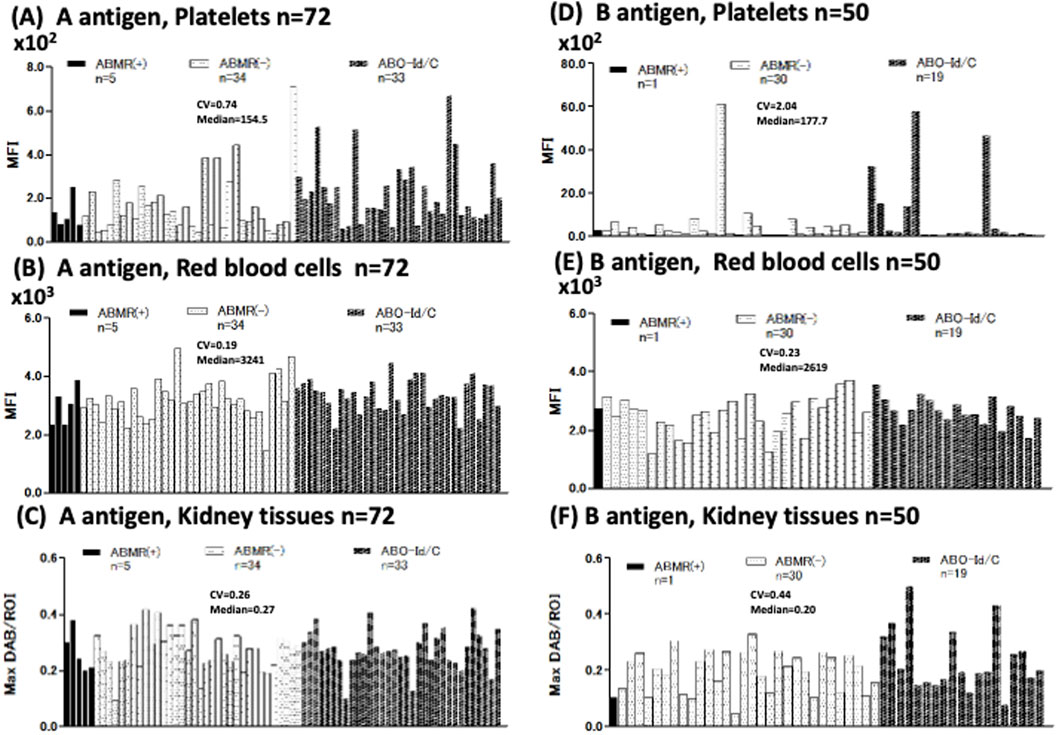
Figure 2. Individual differences in blood group A/B antigen expression in platelets (Plt), red blood cell (RBC), and kidney tissue (Kt). Blood group A antigen expression of Plt, RBC, and Kt (A–C) Antigen A expression of 72 donors [ABMR(+) n = 5, ABMR(−) n = 34, ABO-Id/C n = 33]. Coefficient of variation (CV), index for comparing individual differences. Individual differences in Plt’s antigen A expression were higher than in RBC and Kt (Plt, RBC, and Kt CV = 0.74, 0.19, and 0.26, respectively). B antigen expression of Plt, RBC, and Kt (D–F). B antigen expression of 50 donors [ABMR(+) n = 1, ABMR(−) n = 30, ABO-Id/C n = 19]. Individual differences in Plt’s B antigen expression were strikingly higher than in RBC and Kt (Plt, RBC, and Kt CV were 2.04, 0.23, and 0.44, respectively).
Expression Levels of Blood Type A Antigen of Plt, RBC, and Kt in ABMR and Non-ABMR Groups
Next, we compared A antigen expression in the Plt, RBC, and Kt of ABO-I donors between ABMR and non-ABMR groups. No significant difference in A antigen expression levels was observed between groups (Figure 3). Regarding B antigen expression, although statistical analysis could not be performed because of the very small number of patients with ABMR, no increasing tendency was observed in B antigen expression levels in the ABMR group (Figures 2D–F).
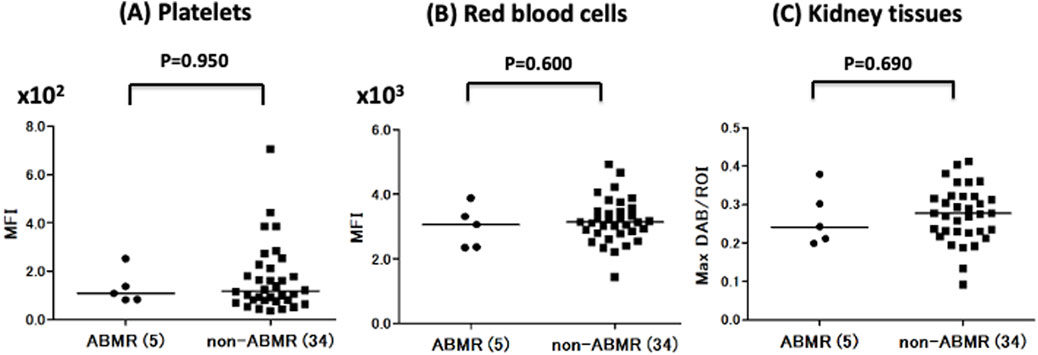
Figure 3. Comparison of Plt, RBC, and Kt’s blood group A antigen expression levels in the antibody-mediated rejection (ABMR) and non-ABMR groups. There were no significant differences in antigen A expression in Plt (A), RBC (B), and Kt (C) between the ABMR group and non-ABMR group.
Anti-A Total IgG and IgM Titers in ABMR and Non-ABMR Groups
Anti-A antibody median total IgG titers were higher in the ABMR group than in the non-ABMR [MFI: 6.59 × 104 (25th–75th percentile, 3.08 × 104–11.9 × 104) vs. MFI: 1.53 × 104 (25th–75th percentile, 1.01 × 104–7.13 × 104; p = 0.110)], as were anti-A antibody total IgM median titers [MFI: 3.35 × 104 (25th–75th percentile, 1.91 × 104–6.91 × 104) vs. MFI: 1.96 × 104 (25th–75th percentile, 1.15 × 104–3.74 × 104; p = 0.175)] (Figure 4; Table 2). MFI values were normalized to those obtained in normal control serum. The cut-off values were calculated from ROC analysis [anti-A IgG: 2.76 × 104, which is a hemagglutination test equivalent to 64 times, area under the curve (AUC) = 0.664, IgM: 2.89 × 104, which is the hemagglutination test equivalent to 32 times, AUC = 0.639] (Table 2; Supplementary Figure S3). Statistical analysis of anti-B titers was not possible due to the small number of ABMR patients.
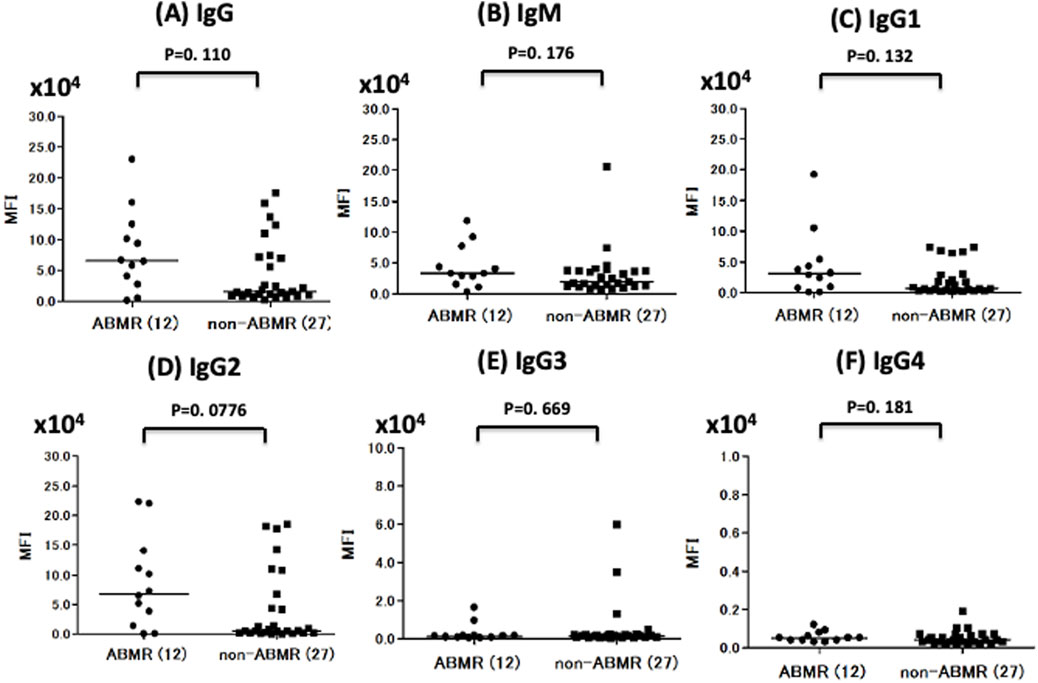
Figure 4. Comparison of anti-A IgG, IgM, and IgG subclass titer in ABMR and non-ABMR groups. The anti-A antibody total IgG median titer (MFI) was higher in the ABMR group than in the non-ABMR (p = 0.110). (A) The anti-A antibody total IgM MFI was higher in the ABMR group than in the non-ABMR (p = 0.175). (B) In anti-A, IgG1 and IgG2 had no significant difference between ABMR group and non-ABMR group. [IgG1: p = 0.131, IgG2: p = 0.077, IgG3: p = 0.669, IgG4 = 0.180; (C–F)].
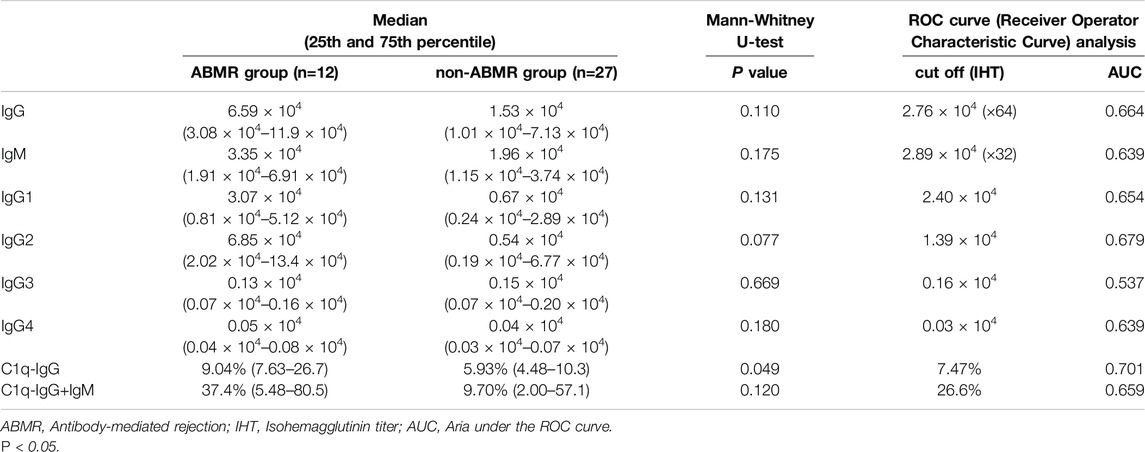
Table 2. Comparison of the patients with anti A IgG, IgM, IgG subclass and C1q titer of ABMR and non-ABMR group in ABO-I.
Anti-A IgG Subclass Distribution in ABMR and Non-ABMR Groups
The anti-A antibody IgG1, IgG2, IgG3, and IgG4 levels were not significantly higher in the ABMR group than in the non-ABMR group [MFI: 3.07 × 104 (25th–75th percentile, 0.81 × 104–5.12 × 104) vs. MFI: 0.67 × 104 (25th–75th percentile, 0.24 × 104–2.89 × 104; p = 0.131 in IgG1], [MFI: 6.85 × 104 (25th–75th percentile, 2.02 × 104–13.4 × 104) vs. MFI: 0.54 × 104 (25th–75th percentile, 0.19 × 104–6.77 × 104), p = 0.077 in IgG2], [MFI: 0.13 × 104 (25th–75th percentile, 0.07 × 104–0.16 × 104) vs. MFI: 0.15 × 104 (25th–75th percentile, 0.07 × 104–0.20 × 104), p = 0.669 in IgG3], [MFI: 0.05 × 104 (25th–75th percentile, 0.04 × 104–0.09 × 104) vs. MFI: 0.04 × 104 (25th–75th percentile, 0.04 × 104–0.08 × 104), p = 0.180 in IgG4], (Figure 4; Table 2). The MFI cut-off values were calculated from ROC analysis (IgG1: 2.40 × 104, AUC = 0.654, IgG2: 1.39 × 104, AUC = 0.679, IgG3: 0.16 × 104, AUC = 0.537, IgG4: 0.03 × 104, AUC = 0.639: Table 2).
C1q Binding Ability to Anti-A Antibody in ABMR and Non-ABMR Groups
C1q binding ability was measured under C1q−IgG and C1q−IgG+IgM (Figure 5). The positivity rates of C1q binding to anti-A antibody were compared between ABMR and non-ABMR groups. C1q−IgG positivity rates were significantly higher in the ABMR group than in the non-ABMR group [DTT-treated C1q, 9.04% (25th–75th percentile, 7.63–26.7) vs. 5.93 (25th–75th percentile, 4.48–10.3), p = 0.049 in anti-A (Figure 5A; Table 2)], as were C1q−IgG+IgM positivity rates [DTT-non-treated C1q, 37.4% (25th–75th percentile, 5.48–80.5) vs. 9.70 (25th–75th percentile, 2.00–57.1), p = 0.120 in anti-A (Figure 5B; Table 2)]. The MFI cut-off values were calculated from ROC analysis (C1q−IgG: 7.47% AUC = 0.701, C1q: 26.6%, AUC = 0.659; Table 2).
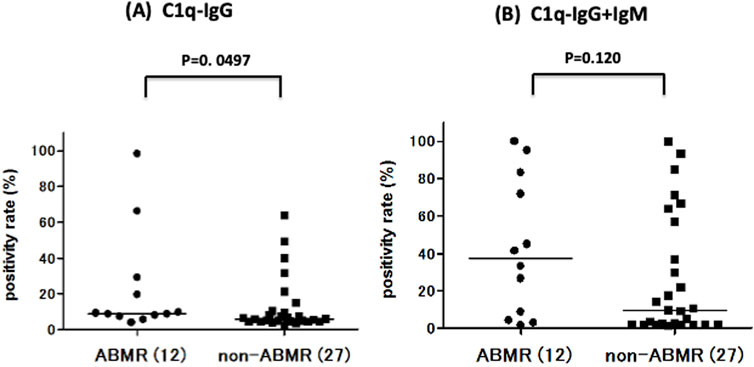
Figure 5. Comparison of anti-A complement C1q titer between ABMR and non-ABMR groups. There was a significant difference between groups in C1q, after removing the influence of IgM binding by DTT, there was a significant difference between groups in anti-A C1q−IgG positive rate (%) [anti-A: p = 0.049; (A)] in C1q−IgG+IgM positive rate (%) [anti-A: p = 0.120; (B)].
Discussion
ABO(H) antigens are oligosaccharides expressed as glycoproteins or glycolipids on cells and tissues, synthesized by glycosyltransferase from different precursor chains based on subtype-1,2,3,4 glycans in humans, depending on the type of cell or tissue [28]. Jeyakatanthan et al. reported differential subtype antigen expression between RBC and tissues or organs [15]. In this study, the quantitative analysis of A/B antigen in Kt, RBC, and Plt demonstrated that neither was associated with ABMR, despite the large inter-individual differences observed in Plt. Ogasawara et al. reported that 7% of Japanese had high A and B antigen expression on Plt [9], and Curtis et al. also found that 7% and 4% of Caucasians showed high A and B antigen expression on Plt, respectively [10]. However, our data did not show a positive correlation between high A/B antigen expression on Plt and ABMR.
The origin of anti-A/B antibodies is still controversial, but the natural antibodies appearing in the neonatal period (3–6 months) are IgMs [29, 30]. Although natural antibodies are usually produced in the absence of exogenous antigens, adult humans have anti-A/B antibodies of the IgG and IgA types produced by sensitization to food, bacteria and viruses which have similar antigens to those of A/B antigens [31]. ABO antigens are glycoprotein antigens, unlike HLA protein antigens. In general, protein antigens promote IgG1 and IgG3 production in B cells, after activation by T cells, whereas glycoprotein antigens mostly promote IgG2 and IgG4 production by B cells in the absence of T cells [32, 33]. The strength of complement activation varies by IgG subclass [18, 19]. IgG1 and IgG3 have a strong affinity for C1q, the first component of the complement pathway, and can thereby activate the complement [34]. Although IgG2 has a weaker complement activation ability than IgG1 and IgG3, the induction of complement activation depends on the density of antigen and antibody [19]. Therefore, high antibody titers of IgG2 can also activate the complement. It is also not yet clear which isotype (IgG or IgM) is more clinically important in ABO-I [35–37]. In the present study, we examined the total IgG/IgM, IgG subclass, and C1q binding ability to IgG/IgG+IgM ABO antibodies in the serum of patients undergoing pre-desensitization therapy. Higher IgG levels were more likely to be a risk factor for acute ABMR than IgM, but there was no significant difference between ABMR and non-ABMR groups. There was also a trend among IgG subclasses toward higher IgG1 and IgG2 levels being risk factors for ABMR, but there was no significant difference among subclasses between ABMR and non-ABMR groups. Comparatively, C1q binding ability (C1q-IgG) is likely to be a marker for ABMR, given the significant differences between ABMR and non-ABMR groups. The C1q binding ability to anti-A antibodies may reflect the density of IgG1 and IgG2 antibodies bound to ABO antigens. Schaub et al. reported that the C1q binding ability to HLA antibodies only reflects the density of bound antibodies and not the composition of IgG subclasses (IgG1-IgG4) [38].
The slightly worse graft engraftment rate of ABO-I compared to ABO-Id/C might be due to side effects such as infection and malignancy or cardiovascular disease [6]. Moreover, renal transplant recipients receiving RIT therapy are less likely to produce antibodies against SARS-Cov-2 [39]. Therefore, introduction of RIT-avoidance (free) protocol may be preferable and could be considered in a certain group [40, 41]. To safely implement such a protocol, we analyzed the association between the C1q binding ability and ABMR, and showed a possibility that C1q binding ability might be a useful marker for RIT avoidance (reduction).
This study has some limitations, including its cross-sectional design (one-point test) which does not allow analyzing changes over time; in addition, there was heterogeneity in immunosuppressive therapy. Nevertheless, this study has two strengths. First, we conducted analysis of antigen expression levels on donors. Second, a complement binding assay, used for detailed examination of HLA antibodies, could be applied to anti-A/B antibodies as well, even if a DTT treatment was necessary to remove the influence of anti-A/B IgM antibodies.
In conclusion, although the amount of A/B antigen in donors cannot explain ABMR in ABO-I, C1q binding ability could be a risk factor for ABMR. Further prospective studies are needed to justify a reduction in desensitization therapy.
Data Availability Statement
The raw data supporting the conclusions of this article will be made available by the authors, without undue reservation.
Ethics Statement
The studies involving humans were approved by The hospital’s institutional ethical committee of Aichi Medical University School of Medicine (authorization number 15-092, 15-H072). The studies were conducted in accordance with the local legislation and institutional requirements. The participants provided their written informed consent to participate in this study. Written informed consent was obtained from the individual(s) for the publication of any potentially identifiable images or data included in this article.
Author Contributions
YM, Participated in research design and collecting data, performance, data analysis and writing of the article. KI, Participated in data analysis and interpretation. KM, Participated in statistic analyzing the data. MO, Participated in collecting data and review of the article. TN, Participated in data analysis and interpretation. YW, Participated in collecting data and review of the article. AT, Participated in pathological analysis. MS, Participated in data analysis and interpretation. SA, Participated in data analysis and interpretation. KI, Participated in data analysis and review of the article. SM, Participated in pathological analysis. TK, Participated in research design and data interpretation, review of the article and writing of the article. All authors contributed to the article and approved the submitted version.
Funding
The author(s) declare financial support was received for the research, authorship, and/or publication of this article. This work was partly supported by a Grant-in-Aid for Scientific Research (No. 16K11063) from the Japan Society for the Promotion of Science (KAKENHI).
Conflict of Interest
The authors declare that the research was conducted in the absence of any commercial or financial relationships that could be construed as a potential conflict of interest.
Acknowledgments
The authors thank Ms. Yuriko Sawa for her excellent pathologic technical assistance. We gratefully thank institute of comprehensive medical research promotion, Aichi Medical University for the flow cytometry measurements. We wish to acknowledge Division for Medical Research Engineering, Nagoya University Graduate School of Medicine, for analysis with FACS Canto II High Throughput Sampler option and Tissuemorph DP. The authors would like to thank Ms. Yuko Matsuzaki for her excellent illustrations. The authors would also like to thank MARUZEN-YUSHODO Co., Ltd. (https://kw.maruzen.co.jp/kousei-honyaku/) for the English language editing.
Supplementary Material
The Supplementary Material for this article can be found online at: https://www.frontierspartnerships.org/articles/10.3389/ti.2024.13407/full#supplementary-material
Supplementary Data 1 | Measuring of the DAB stain area of A/B antigens using the image analysis software Tissuemorph DP. The anti-A/B antibodies were diluted (dilution magnification ranged from ×25 to ×800) and compared in each sample with the highest DAB/ROI value.
Supplementary Data 2 | Calculation of the MFI cut-off values of anti-A IgG, IgM, IgG subclasses antibody and C1q-binding-ability by ROC analysis.
Supplementary Data 3 | Correlation between hemagglutinin titers and MFI of anti-A antibody IgG and IgM.
Abbreviations
ABO-I, ABO blood group incompatible kidney transplantation; ABO-Id/C, ABO blood group identical or compatible kidney transplantation; ABMR, Antibody-mediated rejection; AR, Acute rejection; AUC, Area under the ROC curve; CV, Coefficient of variation; DSA, Donor specific HLA antibody; EC, Endothelial cells; IHT, Isohemagglutinin titer; IST, Immunosuppressive therapy; Kt, Kidney tissues; MFI, Mean fluorescence intensity; NoR/S, Neither rituximab nor splenectomy; Plt, Platelets; RBC, Red blood cells; RIT, Rituximab; ROC, Receiver operating characteristic; ROI, Region of interest; SPX, Splenectomy.
References
1. Lo, P, Sharma, A, Craig, JC, Wyburn, K, Lim, W, Chapman, JR, et al. Preconditioning Therapy in ABO-Incompatible Living Kidney Transplantation: A Systematic Review and Meta-Analysis. Transplantation (2016) 100(4):933–42. doi:10.1097/TP.0000000000000933
2. Takahashi, K, Saito, K, Takahara, S, Fuchinoue, S, Yagisawa, T, Aikawa, A, et al. Results of a Multicenter Prospective Clinical Study in Japan for Evaluating Efficacy and Safety of Desensitization Protocol Based on Rituximab in ABO-Incompatible Kidney Transplantation. Clin Exp Nephrol (2017) 21(4):705–13. doi:10.1007/s10157-016-1321-5
3. Becker, LE, Siebert, D, Süsal, C, Opelz, G, Leo, A, Waldherr, R, et al. Outcomes Following ABO-Incompatible Kidney Transplantation Performed After Desensitization by Nonantigen-Specific Immunoadsorption. Transplantation (2015) 99(11):2364–71. doi:10.1097/TP.0000000000000753
4. Opelz, G, Morath, C, Süsal, C, Tran, TH, Zeier, M, and Döhler, B. Three-Year Outcomes Following 1420 ABO-Incompatible Living-Donor Kidney Transplants Performed After ABO Antibody Reduction: Results From 101 Centers. Transplantation (2015) 99(2):400–4. doi:10.1097/TP.0000000000000312
5. Okada, M, Watarai, Y, Iwasaki, K, Murotani, K, Futamura, K, Yamamoto, T, et al. Favorable Results in ABO-Incompatible Renal Transplantation without B Cell-Targeted Therapy: Advantages and Disadvantages of Rituximab Pretreatment. Clin Transpl (2017) 31(10). doi:10.1111/ctr.13071
6. Charmetant, X, Espi, M, Barba, T, Ovize, A, Morelon, E, Mathieu, C, et al. Predictive Factors of a Viral Neutralizing Humoral Response After a Third Dose of COVID-19 mRNA Vaccine. Am J Transpl (2022) 22(5):1442–50. doi:10.1111/ajt.16990
7. Grupper, A, and Katchman, H. Reduced Humoral Response to mRNA SARS-CoV-2 BNT162b2 Vaccine in Kidney Transplant Recipients Without Prior Exposure to the Virus: Not Alarming, But Should Be Taken Gravely. Am J Transpl (2021) 21(8):2909. doi:10.1111/ajt.16710
8. Moor, MB, Suter-Riniker, F, Horn, MP, Aeberli, D, Amsler, J, Möller, B, et al. Humoral and Cellular Responses to mRNA Vaccines Against SARS-CoV-2 in Patients With a History of CD20 B-Cell-Depleting Therapy (RituxiVac): An Investigator-Initiated, Single-Centre, Open-Label Study. Lancet Rheumatol (2021) 3(11):e789–e797. doi:10.1016/S2665-9913(21)00251-4
9. Ogasawara, K, Ueki, J, Takenaka, M, and Furihata, K. Study on the Expression of ABH Antigens on Platelets. Blood (1993) 82(3):993–9. doi:10.1182/blood.v82.3.993.bloodjournal823993
10. Curtis, BR, Edwards, JT, Hessner, MJ, Klein, JP, and Aster, RH. Blood Group A and B Antigens Are Strongly Expressed on Platelets of Some Individuals. Blood (2000) 96(4):1574–81. doi:10.1182/blood.v96.4.1574
11. Cooling, LL, Kelly, K, Barton, J, Hwang, D, Koerner, TA, and Olson, JD. Determinants of ABH Expression on Human Blood Platelets. Blood (2005) 105(8):3356–64. doi:10.1182/blood-2004-08-3080
12. Sant'Anna Gomes, BM, Estalote, AC, Palatnik, M, Pimenta, G, Pereira, BB, and Do Nascimento, EM. Prevalence, Serologic and Genetic Studies of High Expressers of the Blood Group A Antigen on Platelets. Transfus Med (2010) 20(5):303–14. doi:10.1111/j.1365-3148.2010.01017.x
13. Tasaki, M, Yoshida, Y, Miyamoto, M, Nameta, M, Cuellar, LM, Xu, B, et al. Identification and Characterization of Major Proteins Carrying ABO Blood Group Antigens in the Human Kidney. Transplantation (2009) 87(8):1125–33. doi:10.1097/TP.0b013e31819e0054
14. Lindberg, L, Johansson, SM, Liu, J, Grufman, P, and Holgersson, J. Is There a Clinical Need for a Diagnostic Test Allowing Detection of Chain Type-specific Anti-A and Anti-B? Transfusion (2011) 51(3):494–503. doi:10.1111/j.1537-2995.2010.02870.x
15. Jeyakanthan, M, Tao, K, Zou, L, Meloncelli, PJ, Lowary, TL, Suzuki, K, et al. Chemical Basis for Qualitative and Quantitative Differences Between ABO Blood Groups and Subgroups: Implications for Organ Transplantation. Am J Transpl (2015) 15(10):2602–15. doi:10.1111/ajt.13328
16. Jeyakanthan, M, Meloncelli, PJ, Zou, L, Lowary, TL, Larsen, I, Maier, S, et al. ABH-Glycan Microarray Characterizes ABO Subtype Antibodies: Fine Specificity of Immune Tolerance After ABO-Incompatible Transplantation. Am J Transpl (2016) 16(5):1548–58. doi:10.1111/ajt.13625
17. Tasaki, M, Saito, K, Nakagawa, Y, Imai, N, Ito, Y, Aoki, T, et al. Acquired Downregulation of Donor-Specific Antibody Production After ABO-Incompatible Kidney Transplantation. Am J Transpl (2017) 17(1):115–28. doi:10.1111/ajt.13937
18. Burton, DR, Gregory, L, and Jefferis, R. Aspects of the Molecular Structure of IgG Subclasses. Monogr Allergy (1986) 19:7–35.
19. Garred, P, Michaelsen, TE, and Aase, A. The IgG Subclass Pattern of Complement Activation Depends on Epitope Density and Antibody and Complement Concentration. Scand J Immunol (1989) 30(3):379–82. doi:10.1111/j.1365-3083.1989.tb01225.x
20. Stussi, G, Huggel, K, Lutz, HU, Schanz, U, Rieben, R, and Seebach, JD. Isotype-Specific Detection of ABO Blood Group Antibodies Using a Novel Flow Cytometric Method. Br J Haematol (2005) 130(6):954–63. doi:10.1111/j.1365-2141.2005.05705.x
21. Freitas, MC, Rebellato, LM, Ozawa, M, Nguyen, A, Sasaki, N, Everly, M, et al. The Role of Immunoglobulin-G Subclasses and C1q in De Novo HLA-DQ Donor-Specific Antibody Kidney Transplantation Outcomes. Transplantation (2013) 95(9):1113–9. doi:10.1097/TP.0b013e3182888db6
22. Cicciarelli, JC, Lemp, NA, Chang, Y, Koss, M, Hacke, K, Kasahara, N, et al. Renal Transplant Patients Biopsied for Cause and Tested for C4d, DSA, and IgG Subclasses and C1q: Which Humoral Markers Improve Diagnosis and Outcomes? J Immunol Res (2017) 2017:1652931. doi:10.1155/2017/1652931
23. Valenzuela, NM, and Schaub, S. The Biology of IgG Subclasses and Their Clinical Relevance to Transplantation. Transplantation (2018) 102(1S Suppl. 1):S7-S13–S13. doi:10.1097/TP.0000000000001816
24. Yabu, JM, Higgins, JP, Chen, G, Sequeira, F, Busque, S, and Tyan, DB. C1q-Fixing Human Leukocyte Antigen Antibodies Are Specific for Predicting Transplant Glomerulopathy and Late Graft Failure After Kidney Transplantation. Transplantation (2011) 91(3):342–7. doi:10.1097/TP.0b013e318203fd26
25. Lan, JH, and Tinckam, K. Clinical Utility of Complement Dependent Assays in Kidney Transplantation. Transplantation (2018) 102(1S Suppl. 1):S14-S22–S22. doi:10.1097/TP.0000000000001819
26. Maeda, I, Abe, K, Koizumi, H, Nakajima, C, Tajima, S, Aoki, H, et al. Comparison Between Ki67 Labeling Index Determined Using Image Analysis Software With Virtual Slide System and that Determined Visually in Breast Cancer. Breast Cancer (2016) 23(5):745–51. doi:10.1007/s12282-015-0634-7
27. Kobayashi, T, and Saito, K. A Series of Surveys on Assay for Anti-A/B Antibody by Japanese ABO-Incompatible Transplantation Committee. Xenotransplantation (2006) 13(2):136–40. doi:10.1111/j.1399-3089.2006.00296.x
28. Clausen, H, and Hakomori, S. ABH and Related Histo-Blood Group Antigens; Immunochemical Differences in Carrier Isotypes and Their Distribution. Vox Sang (1989) 56(1):1–20. doi:10.1111/j.1423-0410.1989.tb03040.x
29. Merbl, Y, Zucker-Toledano, M, Quintana, FJ, and Cohen, IR. Newborn Humans Manifest Autoantibodies to Defined Self Molecules Detected by Antigen Microarray Informatics. J Clin Invest (2007) 117(3):712–8. doi:10.1172/JCI29943
30. Bikhet, M, Morsi, M, Hara, H, Rhodes, LA, Carlo, WF, Cleveland, D, et al. The Immune System in Infants: Relevance to Xenotransplantation. Pediatr Transpl (2020) 24(7):e13795. doi:10.1111/petr.13795
31. Springer, GF, and Horton, RE. Blood Group Isoantibody Stimulation in Man by Feeding Blood Group-Active Bacteria. J Clin Invest (1969) 48(7):1280–91. doi:10.1172/JCI106094
32. Wuttke, NJ, Macardle, PJ, and Zola, H. Blood Group Antibodies Are Made by CD5+ and by CD5- B Cells. Immunol Cell Biol. (1997) 75(5):478–83. doi:10.1038/icb.1997.74
33. Ishida, H, Tanabe, K, Ishizuka, T, Furusawa, M, Miyamoto, N, Ishikawa, N, et al. Differences in Humoral Immunity Between a Non-Rejection Group and a Rejection Group After ABO-Incompatible Renal Transplantation. Transplantation (2006) 81(5):665–71. doi:10.1097/01.tp.0000185193.77929.96
34. Schroeder, HW, and Cavacini, L. Structure and Function of Immunoglobulins. J Allergy Clin Immunol (2010) 125(2 Suppl. 2):S41–52. doi:10.1016/j.jaci.2009.09.046
35. Böhmig, GA, Farkas, AM, Eskandary, F, and Wekerle, T. Strategies to Overcome the ABO Barrier in Kidney Transplantation. Nat Rev Nephrol (2015) 11(12):732–47. doi:10.1038/nrneph.2015.144
36. Toki, D, Ishida, H, Setoguchi, K, Shimizu, T, Omoto, K, Shirakawa, H, et al. Acute Antibody-Mediated Rejection in Living ABO-Incompatible Kidney Transplantation: Long-Term Impact and Risk Factors. Am J Transpl (2009) 9(3):567–77. doi:10.1111/j.1600-6143.2008.02538.x
37. Kim, H, Choe, W, Shin, S, Kim, YH, Han, DJ, Park, SK, et al. ABO-Incompatible Kidney Transplantation Can Be Successfully Conducted by Monitoring IgM Isoagglutinin Titers During Desensitization. Transfusion (2020) 60(3):598–606. doi:10.1111/trf.15672
38. Schaub, S, Hönger, G, Koller, MT, Liwski, R, and Amico, P. Determinants of C1q Binding in the Single Antigen Bead Assay. Transplantation (2014) 98(4):387–93. doi:10.1097/TP.0000000000000203
39. Schietzel, S, Anderegg, M, Limacher, A, Born, A, Horn, MP, Maurer, B, et al. Humoral and Cellular Immune Responses on SARS-CoV-2 Vaccines in Patients With Anti-CD20 Therapies: A Systematic Review and Meta-Analysis of 1342 Patients. RMD Open (2022) 8(1):e002036. doi:10.1136/rmdopen-2021-002036
40. Segev, DL, Simpkins, CE, Warren, DS, King, KE, Shirey, RS, Maley, WR, et al. ABO Incompatible High-Titer Renal Transplantation Without Splenectomy or Anti-CD20 Treatment. Am J Transpl (2005) 5(10):2570–5. doi:10.1111/j.1600-6143.2005.01031.x
Keywords: ABO-incompatible kidney transplantation, acute antibody-mediated rejection, A/B antigen expression levels of donor specimens, IgG subclasses, C1q binding ability
Citation: Miwa Y, Iwasaki K, Murotani K, Okada M, Nagasaka T, Watarai Y, Takeda A, Shizuku M, Ashimine S, Ishiyama K, Maruyama S and Kobayashi T (2024) C1q Binding Ability for Prior Risk Assessment of Acute Antibody-Mediated Rejection in ABO-Incompatible Kidney Transplantation. Transpl Int 37:13407. doi: 10.3389/ti.2024.13407
Received: 19 June 2024; Accepted: 30 September 2024;
Published: 15 October 2024.
Copyright © 2024 Miwa, Iwasaki, Murotani, Okada, Nagasaka, Watarai, Takeda, Shizuku, Ashimine, Ishiyama, Maruyama and Kobayashi. This is an open-access article distributed under the terms of the Creative Commons Attribution License (CC BY). The use, distribution or reproduction in other forums is permitted, provided the original author(s) and the copyright owner(s) are credited and that the original publication in this journal is cited, in accordance with accepted academic practice. No use, distribution or reproduction is permitted which does not comply with these terms.
*Correspondence: Yuko Miwa, bWl3YXlAYWljaGktbWVkLXUuYWMuanA=; Takaaki Kobayashi, dGFrYWFraS5rb2JheWFzaGlAYWljaGktbWVkLXUuYWMuanA=