- 1University Hospitals Leuven, Leuven, Belgium
- 2Nephrology and Renal Transplantation Research Group, Department of Microbiology, Immunology and Transplantation, KU Leuven, Leuven, Belgium
- 3Department of Nephrology and Kidney Transplantation, University Hospitals Leuven, Leuven, Belgium
For the past decades, complement activation and complement-mediated destruction of allograft cells were considered to play a central role in anti-HLA antibody-mediated rejection (AMR) of kidney transplants. However, also complement-independent mechanisms are relevant in the downstream immune activation induced by donor-specific antibodies, such as Fc-gamma receptor (FcγR)-mediated direct cellular activation. This article reviews the literature regarding FcγR involvement in AMR, and the potential contribution of FcγR gene polymorphisms to the risk for antibody mediated rejection of kidney transplants. There is large heterogeneity between the studies, both in the definition of the clinical phenotypes and in the technical aspects. The study populations were generally quite small, except for two larger study cohorts, which obviates drawing firm conclusions regarding the associations between AMR and specific FcγR polymorphisms. Although FcγR are central in the pathophysiology of AMR, it remains difficult to identify genetic risk factors for AMR in the recipient’s genome, independent of clinical risk factors, independent of the donor-recipient genetic mismatch, and in the presence of powerful immunosuppressive agents. There is a need for larger, multi-center studies with standardised methods and endpoints to identify potentially relevant FcγR gene polymorphisms that represent an increased risk for AMR after kidney transplantation.
Introduction
Kidney transplantation remains the most cost-effective treatment for patients with end-stage kidney failure (1). Antibody-mediated rejection (AMR) has been identified as a main reason for this failure (2–5). The term “AMR” defines allograft rejections caused by donor-specific antibodies (DSAs), either against anti-human leukocyte antigens (HLA), blood group antigens, or endothelial cell antigens (6). AMR has been reported to occur in 3%–12% of kidney transplant patients (7) but can be as high as 50% in patients with HLA incompatible transplants (8–10).
Complement-mediated destruction of allograft cells induced by donor-specific anti-HLA antibodies (DSAs) is considered a key component to this pathophysiology of AMR, next to other mechanisms including alternative pathways of NK cell activation and antibody-dependent cellular cytotoxicity (11, 12). C1q binds to the antigen-antibody complexes on the graft endothelium. This activates the complement cascade which ultimately produces a membrane attack complex, initiating osmotic cell lysis. One of the complement split proteins (C4d) can covalently bind to the endothelium or basement membrane collagen. The presence of C4d in the allograft biopsy is therefore regarded as a marker of recent complement activation (13).
However, it was illustrated that graft survival is also impaired in patients with DSAs that are not complement-binding, when compared to patients without antibodies (14, 15). Furthermore, complement-inhibiting therapies did not effectively prevent AMR in all patients with non-complement binding DSAs (16–18). Finally, AMR cases often have no microvascular C4d deposition (19). Taken together, these findings suggest a role of complement-independent processes in antibody-mediated damage of kidney allografts.
Antibodies can also lyse target cells by complement-independent pathways, through the IgG Fc portion and FcγRs variably expressed at the surface of natural killer (NK) cells and of monocytes in a process known as antibody-dependent cell-mediated cytotoxicity (ADCC) (20–25). The antibody Fc region can bind to receptors on monocytes, macrophages, neutrophils, and NK cells. Through interaction between the Fc portion of the coating antibody and the Fc gamma receptor on NK cells, a signalling cascade is initiated that results in the release of cytotoxic granules (containing perforin, granzyme B) and production of cytokines (TNF-alpha and IFN-gamma), ultimately inducing apoptosis of the antibody-coated cell (22).
There are both inhibiting and activating FcγRs which differ in IgG affinity and signalling mechanisms. These signalling mechanisms can initiate various effector mechanisms including production of reactive oxygen species, cytokines and cytotoxins, immune cell recruitment and activation (Figure 1). Further evidence through histological appearances of FcγR expressing cells in AMR, transcriptomic signatures of FcγRIIIA transcripts in AMR and genetic association studies in transplantation that show a number of single nucleotide polymorphisms (SNPs) in FcγRs, have led to increasing evidence of the major role that FcγRs play in AMR (26–39).
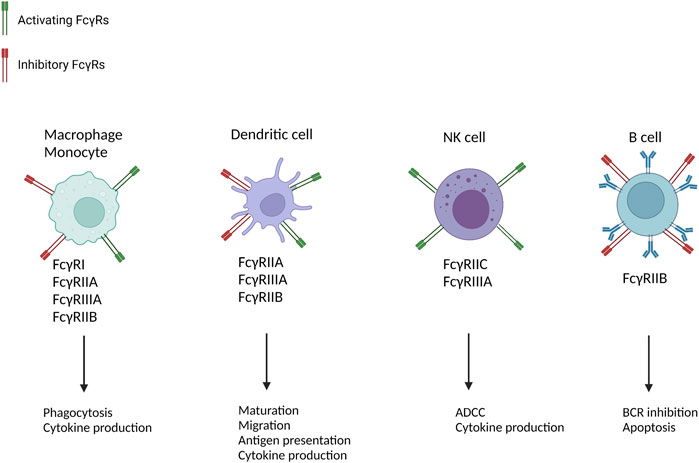
FIGURE 1. Cellular distribution and function of FcγRs [Adapted from Castro-Dopico et al. (41)]. Multiple immune cells are implicated in AMR and express FcγRs. By promoting cell-type-specific immunological mechanisms they contribute to allograft rejection. B-cells only contain the inhibitory FcγRIIB, which is why they lead to BCR inhibition and apoptosis. NK-cells only express activating FcγRs which is why they only lead to activation of immunological mechanisms such as ADCC and cytokine production. Dendritic cells, macrophages and monocytes contain both activating and inhibitory FcγRs. ADCC, antibody-dependent cellular cytotoxicity; ROS, reactive oxygen species; NET, neutrophil extracellular traps; BCR, B-cell receptor.
Most SNPs or genetic polymorphisms have no effect on health or disease development, but some of them can act as biological markers by leading to variations in the amino acid sequence of a gene. This way, certain SNPs can be associated with certain diseases or a predisposition to develop a disease later. Several FcγR gene polymorphisms have been shown to change the functionality of FcγRs (29, 39, 40). Decreased immune cell activation, altered binding characteristics to immunoglobulins and altered receptor functions are some examples of how FcγRs can be influenced by certain SNPs. This article reviews the literature on the role of a complement-independent process via FcγRs in the pathophysiology of AMR, and the possible role of FcγR gene polymorphisms in the risk of rejection, AMR and ADCC. In 2016, Castro-Dopico et al reported on this topic (41). We re-evaluated the literature, including more recent references.
Materials and Methods
A comprehensive literature search was performed by utilizing the following databases: PubMed, Embase and Web of Science core collection.
Our PubMed/MEDLINE search string consisted of the following terms: (“Receptors, IgG”[Mesh] OR “FcγR IIA” [Supplementary Concept] OR “FcγR IIB” [Supplementary Concept] OR “FcγR IIC” [Supplementary Concept] OR “FCΓR3A protein, human” [Supplementary Concept] OR “FCΓR3B protein, human” [Supplementary Concept] OR “FCΓR1A protein, human” [Supplementary Concept] OR “FcγR1 protein, mouse” [Supplementary Concept] OR “FcγR3 protein, mouse” [Supplementary Concept] OR “FcγR2a protein, rat” [Supplementary Concept] OR “Fcγ” OR “Fc gamma” OR “Fcgamma”) AND (“Graft Rejection”[Mesh] OR [(“transplant*” OR “graft*”) AND “reject*”)] AND (“kidney” OR “renal”). 55 hits were found on 14/04/2021.
Our Embase search string consisted of the following terms: “Fc receptor”/exp OR “Fc receptor”: ti, ab, kw OR “Fc receptor IIa”/exp OR “Fc receptor Iib”/exp OR “Fc receptor Iic”: ti, ab, kw OR “fc fragment receptor”: ti, ab, kw OR “FcγR”: ti, ab, kw OR “IgG fc receptor”: ti, ab, kw OR “immunoglobulin fc fragment receptor”: ti, ab, kw OR “immunoglobulin g fc receptor”: ti, ab, kw OR “lymphocyte fc receptor”: ti, ab, kw OR “FcγR”: ti, ab, kw OR “FCΓR1A protein, human”: ti, ab, kw OR “Fc gamma”: ti, ab, kw OR “FCΓR3B protein, human”: ti, ab, kw OR “Fcγ”: ti, ab, kw AND “graft rejection”/exp OR “allograft rejection”: ti, ab, kw OR “graft reaction”: ti, ab, kw OR “allograft reaction”: ti, ab, kw OR “transplant* rejection”: ti, ab, kw AND “kidney”/exp OR “Renal”: ti, ab, kw. 70 hits were found on 07 March 2021.
Our Web of Science core collection search string consisted of the following terms: TS=(“Fc receptor” OR “Fc receptor IIa”/exp OR “Fc receptor IIb”/exp OR “Fc receptor IIc” OR “fc fragment receptor” OR “FcγR” OR “IgG fc receptor” OR “immunoglobulin fc fragment receptor” OR “immunoglobulin g fc receptor” OR “lymphocyte fc receptor” OR “FcγR” OR “FCΓR1A protein, human” OR “Fc gamma” OR “FCΓR3B protein, human” OR “Fcγ”). TS = (“graft rejection” OR “allograft rejection” OR “graft reaction” OR “allograft reaction” OR “transplant* rejection”). TS = (“kidney” OR “Renal”). 47 hits were found on 07 March 2021.
Study Selection
Articles from databases were identified and selected applying subsequent steps:
1) Identification of titles of records through database searching
2) Removal of duplicates
3) Screening and selection of abstracts. Abstracts had to contain information regarding both FcγRs and kidney transplant rejection (preferably AMR).
4) Judgement for eligibility through full-text articles; texts had to contain a thorough description of an FcγR polymorphism and AMR. They needed to report the incidence of the polymorphism comparing kidney transplant recipients with rejection to kidney transplant recipients without rejection.
5) Final inclusion in study.
After careful consideration, only five articles were included in the review. Multiple reviews and other articles were used to provide a framework and to refer to.
Results
Fc-Gamma Receptor and Their Mechanisms of Action
FcγRs are glycoproteins that can be found on the surface of hematopoietic cells and bind to the Fc portion of IgG antibodies. This facilitates a link between the humoral and cellular immune systems (42). The family of FcγRs is involved in antigen presentation, regulation of B cell activation and initiation of intracellular signalling pathways which subsequently lead to immune cell activation and maturation (43). Classical FcγRs include an inhibitory receptor (FcγRIIB) and multiple activating receptors (FcγRI, FcγRIIA, FcγRIIC, FcγRIIIA, and FcγRIIIB).
FcγRs have binding affinity for IgG and can recognize IgG-coated targets, such as opsonized pathogens or immune complexes. After cross-linking of activating FcγRs, tyrosine on the immunoreceptor tyrosine-based activation motif (ITAM) gets phosphorylated. Due note that cross-linking of FcγRs only occurs with aggregated IgG, such as opsonised cells or immune complexes, rather than monomeric IgG (44). Then both Src-kinases Lyn and subsequent recruitment of SH2-containing kinases are responsible for activating ITAM by phosphorylation. ITAMs are located either on the intracellular domain of the FcγRs (e.g., FcγRIIA) or in the associated common γ-chain (e.g., FcγRIIIA). ITAM-P leads to key recruitment of SH2 domain containing kinases, most notably spleen tyrosine kinase (SYK), and the subsequent activation of multiple downstream signalling mediators, including PI3K and PLCγ. All this leads to triggering protein kinase C (PKC) and initiating calcium flux (44, 45). The subsequent mechanisms differ between the different types of immune cells that express FcγR (Figure 1). Differences in these domains account for differences in function of FcyR. In contrast to activating FcγRs, FcγRIIB (inhibitory receptor) contains an intra-cellular immunoreceptor tyrosine-based inhibitory motif (ITIM). Cross-linking of FcγRIIB with activating FcγR leads to Src kinases phosphorylating ITIM and recruiting of inositol phosphatases to neutralise the activating signals (46). Therefore, the FcγRIIB can act as a supplementary regulatory mechanism and suppresses IgG-mediated inflammation (27).
Four different IgG subclasses in humans (IgG1-IgG4) are responsible for the action mechanism of FcγRs. The four IgG subclasses express different affinities to different receptors. IgG1 and IgG3 can efficiently activate the classical route of complement, while IgG2 and IgG4 do this less efficiently or only under certain conditions, as seen with IgG2. This can be explained by the reduced binding of C1q to IgG2 and IgG4 (47).
FcγRs are broadly expressed by hematopoietic cells such as natural killer (NK) cells, mast cells, macrophages, dendritic cells, neutrophils, monocytes, endothelial cells and B-cells (44). Cells can vary in the expression of different types of FcγRs and the levels of expression of these FcγRs, allowing them to modulate the activation threshold when interacting with immune complexes (48). The activation state of FcγR-expressing cells is tightly controlled by the balance between activating and inhibitory FcγR, with the exception of NK cells (49). NK cells express only FcγRIIIA and no inhibitory FcγR. The distribution of the FcγRs across different cell types is illustrated in Figure 1. FcγR-ligated immune cells can directly activate the endothelium by binding to DSA and cause AMR through ADCC without interference of the complement-pathway.
Monocytes/Macrophages
Monocytes are innate immune cells that work as potent phagocytes and that can further differentiate into either macrophages or dendritic cells (50). Several studies suggest that monocyte infiltration is a key component of AMR after transplantation (34, 51, 52).
Macrophages express FcγRIIA, FcγRIIIA and FcγRIIB, with the activating FcγRs being more dominantly expressed. Activation of FcγRs leads to phagocytosis and cytokine release (TNF, IL6, IL-1alpha and neutrophil chemoattractants). These responses are counteracted by the inhibiting FcγRIIB (53). In dendritic cells this inhibiting FcγRIIB is dominantly expressed and suppresses immune-complex-mediated pro-inflammatory cytokine release, T-cell stimulation and migration (54–56).
Neutrophils
Human neutrophils express both FcγRI, FcγRIIA and FcγRIIIB. Activation of FcγRs on neutrophils leads to increased neutrophil adhesion to endothelial cells, cytokine and superoxide production, phagocytosis and neutrophil extracellular trap formation (NETosis) (57–61). When neutrophil infiltration in AMR is present, they are typically found in peritubular capillary lumens (62, 63).
Natural Killer Cells
NK cells primarily express activating FcγRIIIA and in some individuals a small fraction of NK cells may express FcγRIIC (64). As they do not express inhibitory FcγR, they could be the dominant effector cell in ADCC (65). When stimulated through their FcγR, they produce monocyte chemo-attractants CCL3, CCL4 and three effector cytokines; IFN-y, TNF and CSF2 (66).
B-Cells
The inhibitory FcγRIIB is the only FcγR expressed by B-cells. After crosslinking with B-cell receptors, the B-cell activation threshold will increase and suppress further antibody production (27).
Other Cell Types
Eosinophils express FcγRI, FcγRIIA, FcγRIIB and FcγRIIIB. Binding to antibodies induces degranulation. Platelets express FcγRIIA. Mast cells express FcγRIIB and FcγRIIIB. The role of eosinophils, platelets and mast cells seems limited in the process of AMR.
Different Fc-Gamma Receptor Polymorphisms Associated With Antibody-Mediated Rejection
Genetic variation in the genes of human FcγRs can alter receptor expression, function and affinity to IgG (27, 67). FcγR single nucleotide polymorphisms (SNPs) are now considered a hereditary risk factor for infectious and autoimmune diseases (68, 69). Also in allo-immune processes, genetic variations in FcγR genes could lead to different susceptibility to AMR. FcγRI has three non-synonymous SNP mutations (rs7531523, rs12078005, and rs142350980) but no studies investigating the association of these polymorphisms with AMR have been published (70). Furthermore, FcγRIIC has one SNP in intron 7 which has an effect on clearance of parasitaemia, but no studies have been published regarding the link with AMR (71). As there is currently no literature available on their association with AMR, they are not further discussed in this literature review.
FcγRIIA
FcγRIIA is a key FcγR for IgG-mediated responses in macrophages, monocytes or monocyte-derived dendritic cells (3, 72). FcγRIIA can also be found on the surface of neutrophils, platelets, basophils, eosinophils and other cells (73). The FcγRII gene is located on chromosome 1q23. Genetic variation in this gene locus is linked with several autoimmune and inflammatory diseases (68). The best-studied functionally relevant SNP, rs1801274, has been described in the extracellular domain of FcγRIIA, and exchanges adenine (A) to guanine (G) in the coding region in exon 4 of chromosome 1 (q23-24). As a result, histidine (H) is switched into an arginine (R) amino acid at position 131 in the immunoglobulin-like domain (H/R131), leading to altered receptor affinity and specificity (29). In contrast to FcγRIIIA, FcγRIIA polymorphisms seems to have less effect on AMR outcomes. This difference could be explained by the higher affinity of FcγRIIA for IgG1 instead of IgG3, opposite to the affinity observed in FcγRIIIA polymorphisms (74). The lack of inhibitory receptors on NK cells, who primarily express FcγRIIIA and lack inhibitory FcγRIIB expression, could contribute further to this observation (75).
Three studies investigated the association between the allelic frequency of this FcγRIIA H/R131 polymorphism in recipients with stable graft function compared to kidney transplant recipients with rejection (Table 1). First, Pawlik et al. conducted a case-control study in a population of 82 renal transplant recipients and found that the R/R131 genotype was associated with longer graft survival, which they hypothesized to be mechanistically explained by a lower affinity of this FcyR and less cytokine release, leading to a decreased immune response (39). The probability of graft survival over 7 years was 1.74-fold greater among subjects with the R/R131 polymorphism, compared to the H/H131 polymorphism. Next, and in contrast with their previous results, Pawlik et al. conducted another case-control study of 121 renal transplant recipients and found no significant differences in allele frequency between recipients with chronic rejection and recipients with stable graft function (28). However, Yuan et al., showed a significant positive association of the R/R131 genotype with acute kidney rejection (29). When homozygous, higher trends towards acute rejection were also observed. They noted that only 9 out of 46 (20%) non-rejectors had the FcγRIIA homozygote R/R131 polymorphism, compared to 24 out of 53 (45%) rejectors having the R/R131 polymorphism. The frequency of the R/R131 polymorphism was thus significantly higher in the rejector group compared to the non-rejector group. Finally, a recent large multicentre retrospective study with 1,940 kidney transplant recipients, found no association between the FcγRIIA H/R131 polymorphism and death-censored graft survival, graft function or requirement of rejection treatment (76). This study comprised an unselected cohort analysis with a patient cohort derived from the Collaborative Transplant Study (CTS, www.ctstransplant.org).
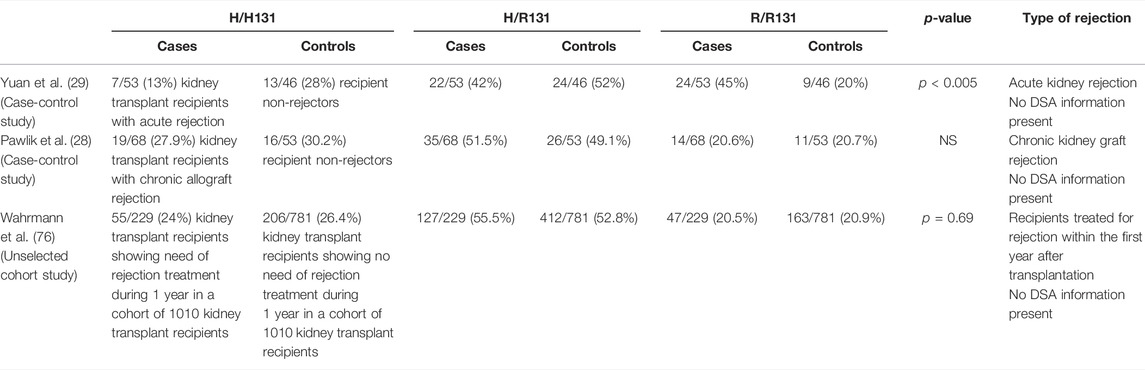
TABLE 1. Distribution of the FcγRIIA genotypes and allele frequencies in patients with vs. without rejection. Numbers are noted as follows: X/Y (%). X = the number of patients with the specific polymorphism; Y = the total number of patients (study recipients or control population); % = the fraction is calculated to the percentage of people who carry the polymorphism; NS = not significant, X = the number of patients with the specific polymorphism. The p-value reflects the significance in differences of the allele frequencies between cases and controls.
FcγRIIIA
FcγIIIA (CD16) is expressed on monocytes/macrophages, dendritic cells, and NK-cells. FcγRIIIA is the only human activating FcγR that has a preferential binding to IgG3. In kidney transplantation, it is suggested that IgG3- DSA positive recipients show more intense microvascular inflammation (77). These findings further suggest the key role of NK cells, monocytes and macrophages in orchestrating the inflammation observed in AMR and may also be, at least in part, the culprits behind the more damaging effects seen with complement-fixing HLA antibodies (15). This further contributes to our hypothesis that different effector mechanisms together lead to graft loss, and not complement-activation alone.
A functional SNP (rs396991) in the gene of FcγRIIIA substitutes a valine (V) to phenylalanine (P) amino acid at position 158 (V/F158), alters the affinity to IgG1 and IgG3 and thus influences immune cell activation (74, 78). For example, Arnold et al. described greater frequency of peritubular capillaritis when the FcγIIIA V158 allele was present due to greater immune cell recruitment in peritubular capillaries (79). Two studies discussed the association between the FcγRIIIA V/F158 polymorphism and AMR after kidney transplantation (Table 2). A case-control study by Litjens et al. linked the V-allele to an increased expression of FcγRIIIA on NK cells and to an increased glomerulitis score in a study of 141 kidney transplant patients (40). Confirming the earlier associations seen in Arnold et al. (79), they observed an association between V-allele and decreased kidney allograft survival after diagnosis of chronic AMR, but the 158V/V genotype itself did not appear to be a risk factor for the development of chronic AMR. Other than the positive association of this polymorphism and increased risk of graft failure after diagnosis of chronic AMR (40), also in heart and lung transplantation clinical associations of cardiac allograft vasculopathy and acute lung transplant rejection with FcγRIIIA polymorphisms have been observed (80, 81). This association between the V/F158 SNP in FcγRIIIA and increased risk of graft failure could be mediated by target cells opsonizing IgG antibodies to bind to FcγRIIIA on immune cells, followed by the release of cytotoxic granules which trigger apoptosis of the target cells. FCGR3A gene expression is also increased in biopsies diagnosed with AMR (36–38). Especially NK cells, which do not express the inhibitory FcγRIIB and thus cannot compensate for overactive FcγRIIIA signalling, could be major contributors to the deleterious effect of this polymorphism.
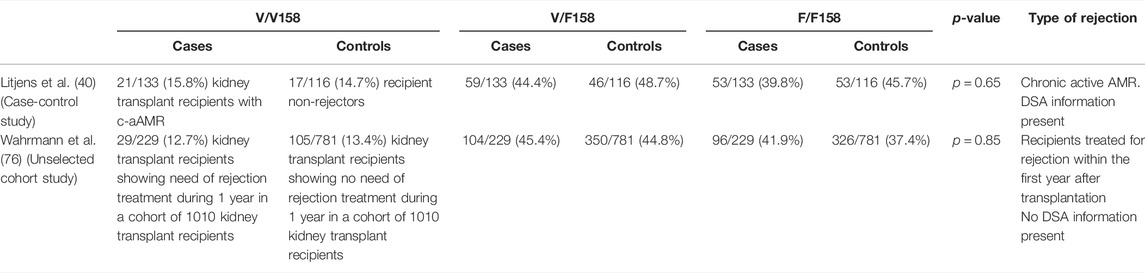
TABLE 2. Distribution of the FcγRIIIA genotypes and allele frequencies in patients with vs. without rejection. Numbers are noted as follows: X/Y (%). X = the number of patients with the specific polymorphism; Y = the total number of patients (study recipients or control population); % = the fraction is calculated to the percentage of people who carry the polymorphism; NS = not significant, X = the number of patients with the specific polymorphism. The p-value reflects the significance in differences of the allele frequencies between cases and controls.
Despite these first suggestions of a significant association between the FcγRIIIA V/F158 polymorphism and AMR and outcome after kidney transplantation, a more recent and larger study included 1940 kidney transplant recipients (76). This study could however not confirm any association of the FcγRIIIA V/F158 polymorphism and impaired allograft function or increased need for rejection treatment within the first year after transplantation. Also in a subanalysis in 438 patients with higher risk of AMR, there was no association of FcγRIIIA polymorphisms with 10-year death-censored graft survival in this subgroup. We do note that Wahrmann et al. didn’t specifically investigate different mechanisms responsible for allograft loss, like microvascular inflammation, whereas Litjens et al. did (40, 76).
FcγRIIIB
FcγRIIIB is expressed on neutrophils and eosinophils. The main function of FcγRIIIB is immune cell clearance of all cells that contain immunoglobulins recognized by FcγRIIIB. By triggering internalisation of captured immune complexes, degradation of antigen-antibody complexes can occur (44). Four amino acid substitutions lead to differences in glycosylation resulting in a FcγRIIIB NA1/NA2 polymorphism. NA1 is more efficient in binding to immune complexes containing IgG1 and IgG3 than NA2 and reduced binding affinity of NA2 genotype could potentially mean that clearance of immune complexes may be reduced (82–85). Furthermore, NA2/NA2 homozygotes show a lower capacity to mediate phagocytosis (86, 87). Because the expression of FcγRIIIB is limited to neutrophils and eosinophils, an association with FcγRIIIB polymorphisms and AMR is not expected. This is due to the fact that neutrophils are rarely observed in late AMR (79). Two studies investigated the difference in incidence of this polymorphism in FcγRIIIB between kidney transplant recipients with stable graft function and kidney transplant recipients with rejection (76, 88) (Table 3). First, a case-control study by Xu et al. showed that NA1/NA2 genotype frequency and allele frequency were not related to acute rejection vs. well-functional grafts in kidney transplant recipients. More recently, Wahrmann et al. confirmed the lack of association between the FcγRIIIB NA1/NA2 polymorphism and death-censored kidney graft survival, graft function or requirement of rejection treatment, in a large cohort of 1,940 kidney transplant recipients.
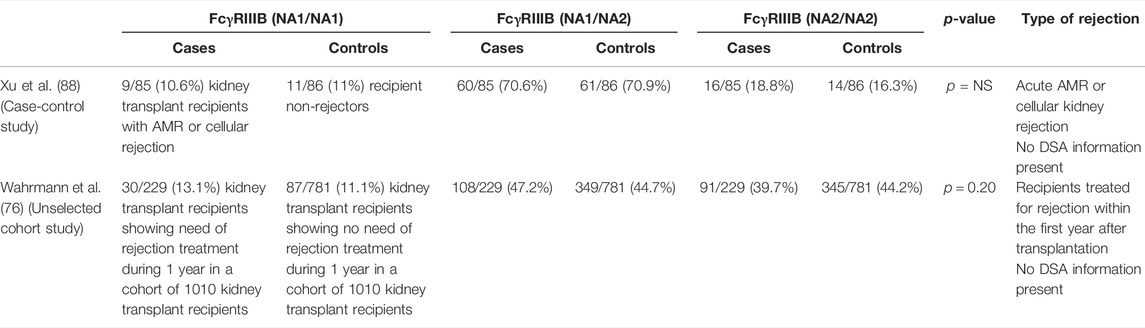
TABLE 3. Distribution of the FcγRIIIB genotypes and allele frequencies in patients with vs. without rejection. Numbers are noted as follows: X/Y (%). X = the number of patients with the specific polymorphism; Y = the total number of patients (study recipients or control population); % = the fraction is calculated to the percentage of people who carry the polymorphism; NS = not significant, X = the number of patients with the specific polymorphism. The p-value reflects the significance in differences of the allele frequencies between cases and controls.
FcγRIIB
FcγRIIB is the only inhibitory FcγR and can be found on B cells, mast cells, macrophages, neutrophils, and eosinophils. The rs1050501 SNP induces a threonine to isoleucine substitution at position 232. Because this occurs within the transmembrane domain of the receptor, FcγRIIB I/T232 is responsible for the dysfunction of the receptor (89, 90). Dysfunction of this inhibitory receptor could theoretically lead to increased immune activation and associations with several autoimmune diseases have been found such as systemic lupus erythematosus, MS and ITP (87, 91–94). Murine studies previously showed associations between FcγRIIB I/T232 and outcomes on kidney allograft by raising the susceptibility to develop chronic AMR (95), but these results could not be replicated in a large human study by Clatworthy et al. (96). They conducted an analysis of three cohorts of 2,851 Caucasian transplant recipients, 570 Afro-Caribbean transplant recipients and 236 patients with a diagnosis of SLE derived from the CTS (96). No association could be found between presence of the FcγRIIB I/T232 polymorphism and differences in 10-year transplant survival. This contradiction could be explained by the observation that expression, structure, associated signalling molecules and most importantly, affinity for different IgG subclasses differ between murine and human FcγRs (97–99). They do however note that their failure to detect an association could be because their effect size of this SNP is smaller than estimated by their power calculations (96). An increased number of patients in a follow-up study could more accurately detect differences or further prove that no associations can be found.
Discussion
Antibody-dependent cellular cytotoxicity is considered to play a major role in the pathophysiology of AMR after kidney transplantation, through the involvement of FcγRs. The mechanism of action and cellular expression of these receptors is well known. Several functional SNPs have been described in these FcγRs and could theoretically impact the risk of AMR after kidney transplantation. Although several studies have addressed this question, it remains however difficult to make conclusions about the role of FcγRs polymorphisms in the risk of AMR. Earlier and smaller studies (28, 30, 40, 88) described associations between FcγR polymorphisms and microcirculation inflammation. However, Wahrmann et al. did not confirm associations between these FcγR gene variants and early rejection, graft function, or long-term allograft failure (76). Even in patients who were sensitised and thus at higher risk for AMR, no associations were found with transplant outcomes.
The discrepancy between the studies are primarily explained by the wide heterogeneity in the choice and definition of the primary endpoints (graft dysfunction, acute and chronic rejection, graft survival time, …), which make comparisons between the studies complex. If for instance the rejection subtype is not evaluated, as was the case for Wahrmann et al. (76), it could be that potential associations between polymorphisms and subtypes of rejection are missed. Other sources of heterogeneity include demographic differences between the cohorts, differences in study design, different background immunological risk of the included patients, numbers of centres, era, etc. Study populations were overall rather small with the exception of the studies by Clatworthy et al. and Wahrmann et al. (76, 96). Also, when AMR is studied, detailed information on DSA is necessary, which is often not available (100). This is a major limitation of the literature on this topic, which importantly hampers making strong conclusions on the association of FcγR polymorphisms and AMR. This could explain why most studies, including Wahrmann et al., have failed to find any associations, while studies where detailed DSA information was available described significant associations between FcγR polymorphisms and the risk of prognosis of AMR. More systematic research on larger-scale collaborative cohorts, and detailed phenotyping of the cases are needed.
In conclusion, our literature review indicates a role of FcγRs in kidney transplant rejection, and the theoretical relevance of the FcγRs polymorphisms in AMR after kidney transplantation. However, in the absence of robust and sufficiently detailed and large-scale studies assessing the actual association of the polymorphisms with well-defined clinical events, we cannot make any robust conclusion on the clinical relevance of these polymorphisms. Furthermore, the two largest, multicenter studies, could not provide evidence for functional FcγR polymorphisms and therefore no impact on incidence of AMR. More systematic large and multi-center studies are needed to robustly determine the potential role of FcγR polymorphisms in the risk of AMR after kidney transplantation, independent of clinical risk factors and the donor-recipient genetic mismatch and in presence of potent immunosuppressive agents, but most importantly, with notion of DSA present.
Author Contributions
BD performed the literature review and drafted the manuscript. EV and MN revised the manuscript critically for important intellectual content. All authors have read and approved the final version of the manuscript.
Conflict of Interest
The authors declare that the research was conducted in the absence of any commercial or financial relationships that could be construed as a potential conflict of interest.
Abbreviations
AMR, antibody-mediated rejection; APC, antigen-presenting cell; DSA, donor-specific antibodies; FcγR, Fc-gamma receptor; IFNγ, interferon gamma; HLA, human leukocyte antigen; ITAM, immunoreceptor tyrosine-based activation motif; ITIM, immunoreceptor tyrosine-based inhibitory motif; MHC, major histocompatibility complex; NK, natural killer; PI3-K, phosphatidylinositol3-kinase; PLCy, phospholipase Cy; PKC, protein kinase C; SNP, single nucleotide polymorphism; TCMR, T-cell mediated rejection.
References
1. Abecassis, M, Bartlett, ST, Collins, AJ, Davis, CL, Delmonico, FL, Friedewald, JJ, et al. Kidney Transplantation as Primary Therapy for End-Stage Renal Disease: A National Kidney Foundation/Kidney Disease Outcomes Quality Initiative (NKF/KDOQI) Conference. Clin J Am Soc Nephrol (2008) 3(2):471–80. doi:10.2215/cjn.05021107
2. Nankivell, BJ, and Kuypers, DR. Diagnosis and Prevention of Chronic Kidney Allograft Loss. Lancet (2011) 378(9800):1428–37. doi:10.1016/S0140-6736(11)60699-5
3. Sellarés, J, de Freitas, DG, Mengel, M, Reeve, J, Einecke, G, Sis, B, et al. Understanding the Causes of Kidney Transplant Failure: The Dominant Role of Antibody-Mediated Rejection and Nonadherence. Am J Transpl (2012) 12(2):388–99. doi:10.1111/j.1600-6143.2011.03840.x
4. van Loon, E, Bernards, J, van Craenenbroeck, AH, and Naesens, M. The Causes of Kidney Allograft Failure: More Than Alloimmunity. A Viewpoint Article. Transplantation (2020) 104(2):E46–56. doi:10.1097/TP.0000000000003012
5. Mayrdorfer, M, Liefeldt, L, Wu, K, Rudolph, B, Zhang, Q, Friedersdorff, F, et al. Exploring the Complexity of Death-Censored Kidney Allograft Failure. J Am Soc Nephrol (2021) 32(6):1513–26. doi:10.1681/asn.2020081215
6. Loupy, A, and Lefaucheur, C. Antibody-Mediated Rejection of Solid-Organ Allografts. N Engl J Med (2018) 379(12):1150–60. doi:10.1056/nejmra1802677
7. Hart, A, Singh, D, Brown, SJ, Wang, JH, and Kasiske, BL. Incidence, Risk Factors, Treatment, and Consequences of Antibody-Mediated Kidney Transplant Rejection: A Systematic Review. Clin Transplant (2021) 35(7):1–12. doi:10.1111/ctr.14320
8. Orandi, BJ, Chow, EHK, Hsu, A, Gupta, N, van Arendonk, KJ, Garonzik-Wang, JM, et al. Quantifying Renal Allograft Loss Following Early Antibody-Mediated Rejection. Am J Transplant (2015) 15(2):489–98. doi:10.1111/ajt.12982
9. Gloor, J, and Stegall, MD. Sensitized Renal Transplant Recipients: Current Protocols and Future Directions. Nat Rev Nephrol (2010) 6(5):297–306. doi:10.1038/nrneph.2010.34
10. Coemans, M, Senev, A, van Loon, E, Lerut, E, Sprangers, B, Kuypers, D, et al. The Evolution of Histological Changes Suggestive of Antibody‐Mediated Injury, in the Presence and Absence of Donor‐Specific anti‐HLA Antibodies. Transpl Int (2021) 34(10):1824–36. doi:10.1111/tri.13964
11. Zhang, R. Donor-Specific Antibodies in Kidney Transplant Recipients. Clin J Am Soc Nephrol (2018) 13(1):182–92. doi:10.2215/cjn.00700117
12. Callemeyn, J, Lamarthée, B, Koenig, A, Koshy, P, Thaunat, O, and Naesens, M. Allorecognition and the Spectrum of Kidney Transplant Rejection. Kidney Int (2022) 101:692–710. doi:10.1016/j.kint.2021.11.029
13. Murata, K, and Baldwin, WM. Mechanisms of Complement Activation, C4d Deposition, and Their Contribution to the Pathogenesis of Antibody-Mediated Rejection. Transplant Rev (2009) 23(3):139–50. doi:10.1016/j.trre.2009.02.005
14. Kauke, T, Oberhauser, C, Lin, V, Coenen, M, Fischereder, M, Dick, A, et al. De Novo Donor-Specific Anti-HLA Antibodies After Kidney Transplantation Are Associated with Impaired Graft Outcome Independently of Their C1q-Binding Ability. Transpl Int (2017) 30(4):360–70. doi:10.1111/tri.12887
15. Loupy, A, Lefaucheur, C, Vernerey, D, Prugger, C, van Huyen, J-PD, Mooney, N, et al. Complement-Binding Anti-HLA Antibodies and Kidney-Allograft Survival. N Engl J Med (2013) 369(13):1215–26. doi:10.1056/nejmoa1302506
16. Lefaucheur, C, Viglietti, D, Hidalgo, LG, Ratner, LE, Bagnasco, SM, Batal, I, et al. Complement-Activating Anti-HLA Antibodies in Kidney Transplantation: Allograft Gene Expression Profiling and Response to Treatment. J Am Soc Nephrol (2018) 29(2):620–35. doi:10.1681/asn.2017050589
17. Montgomery, RA, Orandi, BJ, Racusen, L, Jackson, AM, Garonzik-Wang, JM, Shah, T, et al. Plasma-Derived C1 Esterase Inhibitor for Acute Antibody-Mediated Rejection Following Kidney Transplantation: Results of a Randomized Double-Blind Placebo-Controlled Pilot Study. Am J Transpl (2016) 16(12):3468–78. doi:10.1111/ajt.13871
18. Kulkarni, S, Kirkiles-Smith, NC, Deng, YH, Formica, RN, Moeckel, G, Broecker, V, et al. Eculizumab Therapy for Chronic Antibody-Mediated Injury in Kidney Transplant Recipients: A Pilot Randomized Controlled Trial. Am J Transpl (2017) 17(3):682–91. doi:10.1111/ajt.14001
19. Haas, M, Sis, B, Racusen, LC, Solez, K, Glotz, D, Colvin, RB, et al. Banff 2013 Meeting Report: Inclusion of C4d-Negative Antibody-Mediated Rejection and Antibody-Associated Arterial Lesions. Am J Transplant (2014) 14(2):272–83. doi:10.1111/ajt.12590
20. Farkash, EA, and Colvin, RB. Diagnostic Challenges in Chronic Antibody-Mediated Rejection. Nat Rev Nephrol (2012) 8(5):255–7. doi:10.1038/nrneph.2012.61
21. Zhang, Z-X, Huang, X, Jiang, J, Lau, A, Yin, Z, Liu, W, et al. Natural Killer Cells Mediate Long-Term Kidney Allograft Injury. Transplantation (2015) 99(5):916–24. doi:10.1097/tp.0000000000000665
22. Resch, T, Fabritius, C, Ebner, S, Ritschl, P, and Kotsch, K. The Role of Natural Killer Cells in Humoral Rejection. Transplantation (2015) 99(7):1335–40. doi:10.1097/tp.0000000000000757
23. Crespo, M, Yelamos, J, Redondo, D, Muntasell, A, Perez-Saéz, MJ, López-Montañés, M, et al. Circulating NK-Cell Subsets in Renal Allograft Recipients with Anti-HLA Donor-Specific Antibodies. Am J Transplant (2015) 15(3):806–14. doi:10.1111/ajt.13010
24. Bachelet, T, Couzi, L, Pitard, V, Sicard, X, Rigothier, C, Lepreux, S, et al. Cytomegalovirus-Responsive γδ T Cells: Novel Effector Cells in Antibody-Mediated Kidney Allograft Microcirculation Lesions. J Am Soc Nephrol (2014) 25(11):2471–82. doi:10.1681/asn.2013101052
25. Fishman, JA. What's New and What's Hot? Basic Science at the American Transplant Congress 2012. Am J Transplant (2013) 13(2):275–80. doi:10.1111/ajt.12015
26. Nimmerjahn, F, and Ravetch, JV. Fcγ Receptors as Regulators of Immune Responses. Nat Rev Immunol (2008) 8(1):34–47. doi:10.1038/nri2206
27. Smith, KGC, and Clatworthy, MR. FcγRIIB in Autoimmunity and Infection: Evolutionary and Therapeutic Implications. Nat Rev Immunol (2010) 10(5):328–43. doi:10.1038/nri2762
28. Pawlik, A, Florczak, M, Bak, L, Da̧browska-Zamojcin, E, Rozanski, J, Domanski, L, et al. The FcγRIIa Polymorphism in Patients with Chronic Kidney Graft Rejection. Transplant Proc (2004) 36(5):1311–3. doi:10.1016/j.transproceed.2004.05.076
29. Yuan, FF, Watson, N, Sullivan, JS, Biffin, S, Moses, J, Geczy, AF, et al. Association of Fc Gamma Receptor IIA Polymorphisms with Acute Renal-Allograft Rejection. Transplantation (2004) 78(5):766–9. doi:10.1097/01.tp.0000132560.77496.cb
30. Ozkayin, N, Mir, S, and Afig, B. The Role of Fcγ Receptor Gene Polymorphism in Pediatric Renal Transplant Rejections. Transplant Proc (2008) 40(10):3367–74. doi:10.1016/j.transproceed.2008.08.137
31. Arnold, M-L, Fuernrohr, BG, Weiß, KM, Harre, U, Wiesener, MS, and Spriewald, BM. Association of a Coding Polymorphism in Fc Gamma Receptor 2A and Graft Survival in Re-transplant Candidates. Hum Immunol (2015) 76(10):759–64. doi:10.1016/j.humimm.2015.09.034
32. Sharp, PEH, Martin-Ramirez, J, Mangsbo, SM, Boross, P, Pusey, CD, Touw, IP, et al. FcγRIIb on Myeloid Cells and Intrinsic Renal Cells Rather Than B Cells Protects from Nephrotoxic Nephritis. J Immunol (2013) 190(1):340–8. doi:10.4049/jimmunol.1202250
33. Magil, AB, and Tinckam, K. Monocytes and Peritubular Capillary C4d Deposition in Acute Renal Allograft Rejection. Kidney Int (2003) 63(5):1888–93. doi:10.1046/j.1523-1755.2003.00921.x
34. Tinckam, KJ, Djurdjev, O, and Magil, AB. Glomerular Monocytes Predict Worse Outcomes After Acute Renal Allograft Rejection Independent of C4d Status. Kidney Int (2005) 68(4):1866–74. doi:10.1111/j.1523-1755.2005.00606.x
35. Xu, L, Collins, J, Drachenberg, C, Kukuruga, D, and Burke, A. Increased Macrophage Density of Cardiac Allograft Biopsies Is Associated with Antibody-Mediated Rejection and Alloantibodies to HLA Antigens. Clin Transpl (2014) 28(5):554–60. doi:10.1111/ctr.12348
36. Hidalgo, LG, Sis, B, Sellares, J, Campbell, PM, Mengel, M, Einecke, G, et al. NK Cell Transcripts and NK Cells in Kidney Biopsies from Patients with Donor-Specific Antibodies: Evidence for NK Cell Involvement in Antibody-Mediated Rejection. Am J Transpl (2010) 10(8):1812–22. doi:10.1111/j.1600-6143.2010.03201.x
37. Hidalgo, LG, Sellares, J, Sis, B, Mengel, M, Chang, J, and Halloran, PF. Interpreting NK Cell Transcripts versus T Cell Transcripts in Renal Transplant Biopsies. Am J Transpl (2012) 12(5):1180–91. doi:10.1111/j.1600-6143.2011.03970.x
38. Venner, JM, Hidalgo, LG, Famulski, KS, Chang, J, and Halloran, PF. The Molecular Landscape of Antibody-Mediated Kidney Transplant Rejection: Evidence for NK Involvement Through CD16a Fc Receptors. Am J Transplant (2015) 15(5):1336–48. doi:10.1111/ajt.13115
39. Pawlik, A, Florczak, M, Bąk, L, Dutkiewicz, G, Pudło, A, and Gawronska-Szklarz, B. The Correlation Between FcγRIIA Polymorphism and Renal Allograft Survival. Transplant Proc (2002) 34(8):3138–9. doi:10.1016/s0041-1345(02)03584-4
40. Litjens, N, Peeters, A, Gestel, JK-v., Klepper, M, and Betjes, M. The FCGR3A 158 V/V-genotype Is Associated with Decreased Survival of Renal Allografts with Chronic Active Antibody-Mediated Rejection. Sci Rep (2021) 11(1):7903. doi:10.1038/s41598-021-86943-3
41. Castro-Dopico, T, and Clatworthy, MR. Fcγ Receptors in Solid Organ Transplantation. Curr Transpl Rep (2016) 3(4):284–93. doi:10.1007/s40472-016-0116-7
42. Li, X, Ptacek, TS, Brown, EE, and Edberg, JC. Fcγ Receptors: Structure, Function and Role as Genetic Risk Factors in SLE. Genes Immun (2009) 10(5):380–9. doi:10.1038/gene.2009.35
43. Guilliams, M, Bruhns, P, Saeys, Y, Hammad, H, and Lambrecht, BN. The Function of Fcγ Receptors in Dendritic Cells and Macrophages. Nat Rev Immunol (2014) 14(2):94–108. doi:10.1038/nri3582
44. Junker, F, Gordon, J, and Qureshi, O. Fc Gamma Receptors and Their Role in Antigen Uptake, Presentation, and T Cell Activation. Front Immunol (2020) 11:1393. doi:10.3389/fimmu.2020.01393
45. Daëron, M. Fc Receptor Biology. Annu Rev Immunol (1997) 15:203–34. Available from: http://www.ncbi.nlm.nih.gov/pubmed/9143687.
46. Ono, M, Bolland, S, Tempst, P, and Ravetch, JV. Role of the Inositol Phosphatase SHIP in Negative Regulation of the Immune System by the Receptor FeγRIIB. Nature (1996) 383(6597):263–6. doi:10.1038/383263a0
47. Tao, MH, Smith, RI, and Morrison, SL. Structural Features of Human Immunoglobulin G that Determine Isotype-Specific Differences in Complement Activation. J Exp Med (1993) 178(2):661–7. doi:10.1084/jem.178.2.661
48. Rosales, C. Fcγ Receptor Heterogeneity in Leukocyte Functional Responses. Front Immunol (2017) 8(MAR):1–13. doi:10.3389/fimmu.2017.00280
49. Bryceson, YT, March, ME, Ljunggren, H-G, and Long, EO. Synergy Among Receptors on Resting NK Cells for the Activation of Natural Cytotoxicity and Cytokine Secretion. Blood (2006) 107(1):159–66. doi:10.1182/blood-2005-04-1351
50. Auffray, C, Sieweke, MH, and Geissmann, F. Blood Monocytes: Development, Heterogeneity, and Relationship with Dendritic Cells. Annu Rev Immunol (2009) 27:669–92. doi:10.1146/annurev.immunol.021908.132557
51. Magil, AB, and Magil, AB. Monocytes/Macrophages in Renal Allograft Rejection. Transplant Rev (2009) 23(4):199–208. doi:10.1016/j.trre.2009.06.005
52. Bosch, TPP, Hilbrands, LB, Kraaijeveld, R, Litjens, NHR, Rezaee, F, Nieboer, D, et al. Pretransplant Numbers of CD16 + Monocytes as a Novel Biomarker to Predict Acute Rejection After Kidney Transplantation: A Pilot Study. Am J Transpl (2017) 17(10):2659–67. doi:10.1111/ajt.14280
53. Clatworthy, MR, and Smith, KGC. FcγRIIb Balances Efficient Pathogen Clearance and the Cytokine-Mediated Consequences of Sepsis. J Exp Med (2004) 199(5):717–23. doi:10.1084/jem.20032197
54. Clatworthy, MR, Aronin, CEP, Mathews, RJ, Morgan, NY, Smith, KGC, and Germain, RN. Immune Complexes Stimulate CCR7-Dependent Dendritic Cell Migration to Lymph Nodes. Nat Med (2014) 20(12):1458–63. doi:10.1038/nm.3709
55. Dhodapkar, KM, Banerjee, D, Connolly, J, Kukreja, A, Matayeva, E, Veri, MC, et al. Selective Blockade of the Inhibitory Fcγ Receptor (FcγRIIB) in Human Dendritic Cells and Monocytes Induces a Type I Interferon Response Program. J Exp Med (2007) 204(6):1359–69. doi:10.1084/jem.20062545
56. Dhodapkar, KM, Kaufman, JL, Ehlers, M, Banerjee, DK, Bonvini, E, Koenig, S, et al. Selective Blockade of Inhibitory Fcγ Receptor Enables Human Dendritic Cell Maturation with IL-12p70 Production and Immunity to Antibody-Coated Tumor Cells. Proc Natl Acad Sci U.S.A (2005) 102(8):2910–5. doi:10.1073/pnas.0500014102
57. Rosales, C, and Brown, EJ. Signal Transduction by Neutrophil Immunoglobulin G Fc Receptors. Dissociation of Intracytoplasmic Calcium Concentration Rise from Inositol 1,4,5-Trisphosphate. J Biol Chem (1992) 267(8):5265–71. doi:10.1016/S0021-9258(18)42761-5
58. Min, X, Liu, C, Wei, Y, Wang, N, Yuan, G, Liu, D, et al. Expression and Regulation of Complement Receptors by Human Natural Killer Cells. Immunobiology (2014) 219(9):671–9. doi:10.1016/j.imbio.2014.03.018
59. Coxon, A, Cullere, X, Knight, S, Sethi, S, Wakelin, MW, Stavrakis, G, et al. FcγRIII Mediates Neutrophil Recruitment to Immune Complexes. A Mechanism for Neutrophil Accumulation in Immune-Mediated Inflammation. Immunity (2001) 14(6):693–704. doi:10.1016/s1074-7613(01)00150-9
60. Kessenbrock, K, Krumbholz, M, Schönermarck, U, Back, W, Gross, WL, Werb, Z, et al. Netting Neutrophils in Autoimmune Small-Vessel Vasculitis. Nat Med (2009) 15(6):623–5. doi:10.1038/nm.1959
61. Sur Chowdhury, C, Giaglis, S, Walker, UA, Buser, A, Hahn, S, and Hasler, P. Enhanced Neutrophil Extracellular Trap Generation in Rheumatoid Arthritis: Analysis of Underlying Signal Transduction Pathways and Potential Diagnostic Utility. Arthritis Res Ther (2014) 16(3):R122–14. doi:10.1186/ar4579
62. Halloran, PF, Wadgymar, A, Ritchie, S, Falk, J, Solez, K, and Srinivasa, NS. The Significance of the Anti-Class I Antibody Response: I. Clinical and Pathologic Features of Anti-Class I-Mediated Rejection. Transplantation (1990) 49:85–90. doi:10.1097/00007890-199001000-00019
63. Morozumi, K, Katoh, M, Horike, K, Oikawa, T, Takeuchi, O, Kimura, G, et al. Pathologic Characteristics of Acute Humoral Rejection After ABO-Incompatible Kidney Transplantation. Transpl Proc (2001) 33(7–8):3299–300. doi:10.1016/s0041-1345(01)02401-0
64. Dutertre, C-A, Bonnin-Gélizé, E, Pulford, K, Bourel, D, Fridman, W-H, and Teillaud, J-L. A Novel Subset of NK Cells Expressing High Levels of Inhibitory FcγRIIB Modulating Antibody-Dependent Function. J Leukoc Biol (2008) 84(6):1511–20. doi:10.1189/jlb.0608343
65. Charreau, B. Cellular and Molecular Crosstalk of Graft Endothelial Cells During AMR: Effector Functions and Mechanisms. Transplantation (2021) 105:e156. doi:10.1097/tp.0000000000003741
66. Parkes, MD, Halloran, PF, and Hidalgo, LG. Evidence for CD16a-Mediated NK Cell Stimulation in Antibody-Mediated Kidney Transplant Rejection. Transplantation (2017) 101(4):e102–e111. doi:10.1097/tp.0000000000001586
67. Willcocks, LC, Smith, KGC, and Clatworthy, MR. Low-Affinity Fcγ Receptors, Autoimmunity and Infection. Expert Rev Mol Med (2009) 11:e24. doi:10.1017/s1462399409001161
68. Roederer, M, Quaye, L, Mangino, M, Beddall, MH, Mahnke, Y, Chattopadhyay, P, et al. The Genetic Architecture of the Human Immune System: A Bioresource for Autoimmunity and Disease Pathogenesis. Cell (2015) 161(2):387–403. doi:10.1016/j.cell.2015.02.046
69. Magnusson, V, Johanneson, B, Lima, G, Odeberg, J, Alarcón-Segovia, D, Alarcón-Riquelme, ME, et al. Both Risk Alleles for FcγRIIA and FcγRIIIA Are Susceptibility Factors for SLE: A Unifying Hypothesis. Genes Immun (2004) 5(2):130–7. doi:10.1038/sj.gene.6364052
70. Brandsma, AM, ten Broeke, T, van Dueren den Hollander, E, Caniels, TG, Kardol-Hoefnagel, T, Kuball, J, et al. Single Nucleotide Polymorphisms of the High Affinity IgG Receptor FcγRI Reduce Immune Complex Binding and Downstream Effector Functions. J Immunol (2017) 199(7):2432–9. doi:10.4049/jimmunol.1601929
71. Amiah, MA, Ouattara, A, Okou, DT, N'Guetta, SA, and Yavo, W. Polymorphisms in Fc Gamma Receptors and Susceptibility to Malaria in an Endemic Population. Front Immunol (2020) 11:561142–16. doi:10.3389/fimmu.2020.561142
72. Vogelpoel, LTC, Hansen, IS, Visser, MW, Nagelkerke, SQ, Kuijpers, TW, Kapsenberg, ML, et al. FcγRIIa Cross-Talk with TLRs, IL-1R, and IFNγR Selectively Modulates Cytokine Production in Human Myeloid Cells. Immunobiology (2015) 220(2):193–9. doi:10.1016/j.imbio.2014.07.016
73. Rosenfeld, SI, Looney, RJ, Leddy, JP, Phipps, DC, Abraham, GN, and Anderson, CL. Human Platelet Fc Receptor for Immunoglobulin G. Identification as a 40,000-Molecular-Weight Membrane Protein Shared by Monocytes. J Clin Invest (1985) 76(6):2317–22. doi:10.1172/jci112242
74. Bruhns, P, Iannascoli, B, England, P, Mancardi, DA, Fernandez, N, Jorieux, S, et al. Specificity and Affinity of Human Fcγ Receptors and Their Polymorphic Variants for Human IgG Subclasses. Blood (2009) 113(16):3716–25. doi:10.1182/blood-2008-09-179754
75. Metes, D, Ernst, LK, Chambers, WH, Sulica, A, Herberman, RB, and Morel, PA. Expression of Functional CD32 Molecules on Human NK Cells Is Determined by an Allelic Polymorphism of the FcγRIIC Gene. Blood (1998) 91(7):2369–80. doi:10.1182/blood.v91.7.2369.2369_2369_2380
76. Wahrmann, M, Döhler, B, Arnold, M-L, Scherer, S, Mayer, KA, Haindl, S, et al. Functional Fc Gamma Receptor Gene Polymorphisms and Long-Term Kidney Allograft Survival. Front Immunol (2021) 12:724331. doi:10.3389/fimmu.2021.724331
77. Lefaucheur, C, Viglietti, D, Bentlejewski, C, Duong van Huyen, J-P, Vernerey, D, Aubert, O, et al. IgG Donor-Specific Anti-Human HLA Antibody Subclasses and Kidney Allograft Antibody-Mediated Injury. J Am Soc Nephrol (2016) 27(1):293–304. doi:10.1681/asn.2014111120
78. Wu, J, Edberg, JC, Redecha, PB, Bansal, V, Guyre, PM, Coleman, K, et al. A Novel Polymorphism of FcgammaRIIIa (CD16) Alters Receptor Function and Predisposes to Autoimmune Disease. J Clin Invest (1997) 100(5):1059–70. doi:10.1172/jci119616
79. Arnold, ML, Kainz, A, Hidalgo, LG, Eskandary, F, Kozakowski, N, Wahrmann, M, et al. Functional Fc Gamma Receptor Gene Polymorphisms and Donor-Specific Antibody-Triggered Microcirculation Inflammation. Am J Transpl (2018) 18(9):2261–73. doi:10.1111/ajt.14710
80. Paul, P, Picard, C, Sampol, E, Lyonnet, L, di Cristofaro, J, Paul-Delvaux, L, et al. Genetic and Functional Profiling of CD16-Dependent Natural Killer Activation Identifies Patients at Higher Risk of Cardiac Allograft Vasculopathy. Circulation (2018) 137(10):1049–59. doi:10.1161/circulationaha.117.030435
81. Paul, P, Pedini, P, Lyonnet, L, di Cristofaro, J, Loundou, A, Pelardy, M, et al. FCGR3A and FCGR2A Genotypes Differentially Impact Allograft Rejection and Patients' Survival After Lung Transplant. Front Immunol (2019) 10:1208. doi:10.3389/fimmu.2019.01208
82. Ozturk, C, Aksu, G, Berdeli, A, and Kutukculer, N. Fc Gamma RIIa, IIIa and IIIb Polymorphisms in Turkish Children Susceptible to Recurrent Infectious Diseases. Clin Exper. Med (2006) 6(1):27–32. doi:10.1007/s10238-006-0090-y
83. Salmon, JE, Edberg, JC, Brogle, NL, and Kimberly, RP. Allelic Polymorphisms of Human Fc Gamma Receptor IIA and Fc Gamma Receptor IIIB. Independent Mechanisms for Differences in Human Phagocyte Function. J Clin Invest (1992) 89(4):1274–81. doi:10.1172/jci115712
84. Bredius, RG, Fijen, CA, de Haas, M, Kuijper, EJ, Weening, RS, van de Winkel, JG, et al. Role of Neutrophil Fc Gamma RIIa (CD32) and Fc Gamma RIIIb (CD16) Polymorphic Forms in Phagocytosis of Human IgG1- and IgG3-Opsonized Bacteria and Erythrocytes. Immunology (1994) 83(4):624–30. Available from: http://www.ncbi.nlm.nih.gov/pubmed/7875742.
85. Aitman, TJ, Dong, R, Vyse, TJ, Norsworthy, PJ, Johnson, MD, Smith, J, et al. Copy Number Polymorphism in Fcgr3 Predisposes to Glomerulonephritis in Rats and Humans. Nature (2006) 439(7078):851–5. doi:10.1038/nature04489
86. Hatta, Y, Tsuchiya, N, Ohashi, J, Matsushita, M, Fujiwara, K, Hagiwara, K, et al. Association of Fcγ Receptor IIIB, but Not of Fcγ Receptor IIA and IIIA, Polymorphisms with Systemic Lupus Erythematosus in Japanese. Genes Immun (1999) 1(1):53–60. doi:10.1038/sj.gene.6363639
87. Siriboonrit, U, Tsuchiya, N, Sirikong, M, Kyogoku, C, Bejrachandra, S, Suthipinittharm, P, et al. Association of Fcγ Receptor IIb and IIIb Polymorphisms with Susceptibility to Systemic Lupus Erythematosus in Thais. Tissue Antigens (2003) 61(5):374–83. doi:10.1034/j.1399-0039.2003.00047.x
88. Xu, G, He, Q, Shou, Z, Wang, H, Wang, R, Jiang, H, et al. Association of Fc Gamma Receptor IIIB Polymorphism with Renal-Allogrft in Chinese. Transpl Immunol (2007) 18(1):28–31. doi:10.1016/j.trim.2007.04.002
89. Floto, RA, Clatworthy, MR, Heilbronn, KR, Rosner, DR, MacAry, PA, Rankin, A, et al. Loss of Function of a Lupus-Associated FcγRIIb Polymorphism Through Exclusion from Lipid Rafts. Nat Med (2005) 11(10):1056–8. doi:10.1038/nm1288
90. Kono, H, Kyogoku, C, Suzuki, T, Tsuchiya, N, Honda, H, Yamamoto, K, et al. FcγRIIB Ile232Thr Transmembrane Polymorphism Associated with Human Systemic Lupus Erythematosus Decreases Affinity to Lipid Rafts and Attenuates Inhibitory Effects on B Cell Receptor Signaling. Hum Mol Genet (2005) 14(19):2881–92. doi:10.1093/hmg/ddi320
91. Chu, ZT, Tsuchiya, N, Kyogoku, C, Ohashi, J, Qian, YP, Xu, SB, et al. Association of Fcgamma Receptor IIb Polymorphism with Susceptibility to Systemic Lupus Erythematosus in Chinese: A Common Susceptibility Gene in the Asian Populations. Tissue Antigens (2004) 63(1):21–7. doi:10.1111/j.1399-0039.2004.00142.x
92. Willcocks, LC, Carr, EJ, Niederer, HA, Rayner, TF, Williams, TN, Yang, W, et al. A Defunctioning Polymorphism in FCGR2B Is Associated with Protection Against Malaria but Susceptibility to Systemic Lupus Erythematosus. Proc Natl Acad Sci U.S.A (2010) 107(17):7881–5. doi:10.1073/pnas.0915133107
93. Tackenberg, B, Jelčić, I, Baerenwaldt, A, Oertel, WH, Sommer, N, Nimmerjahn, F, et al. Erratum: Impaired Inhibitory Fcγ Receptor IIB Expression on B Cells in Chronic Inflammatory Demyelinating Polyneuropathy (Proc Natl Acad Sci USA 106 (4788-4792)). Proc Natl Acad Sci U S A (2015) 112(31):E4336. doi:10.1073/pnas.0807319106
94. Bruin, M, Bierings, M, Uiterwaal, C, Révész, T, Bode, L, Wiesman, M-E, et al. Platelet Count, Previous Infection and FCGR2B Genotype Predict Development of Chronic Disease in Newly Diagnosed Idiopathic Thrombocytopenia in Childhood: Results of a Prospective Study. Br J Haematol (2004) 127(5):561–7. doi:10.1111/j.1365-2141.2004.05235.x
95. Callaghan, CJ, Win, TS, Motallebzadeh, R, Conlon, TM, Chhabra, M, Harper, I, et al. Regulation of Allograft Survival by Inhibitory FcγRIIb Signaling. J Immunol (2012) 189(12):5694–702. doi:10.4049/jimmunol.1202084
96. Clatworthy, MR, Matthews, RJ, Doehler, B, Willcocks, LC, Opelz, G, and Smith, KGC. Defunctioning Polymorphism in the Immunoglobulin G Inhibitory Receptor (FcγRIIB-T/T232) Does Not Impact on Kidney Transplant or Recipient Survival. Transplantation (2014) 98(3):285–91. doi:10.1097/tp.0000000000000287
97. Bruhns, P. Properties of Mouse and Human IgG Receptors and Their Contribution to Disease Models. Blood (2012) 119(24):5640–9. doi:10.1182/blood-2012-01-380121
98. Bruhns, P, and Jönsson, F. Mouse and Human FcR Effector Functions. Immunol Rev (2015) 268(1):25–51. doi:10.1111/imr.12350
99. Colucci, F, di Santo, JP, and Leibson, PJ. Natural Killer Cell Activation in Mice and Men: Different Triggers for Similar Weapons? Nat Immunol (2002) 3(9):807–13. doi:10.1038/ni0902-807
100. Schinstock, CA, Askar, M, Bagnasco, SM, Batal, I, Bow, L, Budde, K, et al. A 2020 Banff Antibody-Mediated Injury Working Group Examination of International Practices for Diagnosing Antibody-Mediated Rejection in Kidney Transplantation - a Cohort Study. Transpl Int (2021) 34(3):488–98. doi:10.1111/tri.13813
Keywords: kidney transplant, renal transplantation, antibody-mediated rejection, AMR, FcγR, FcγR polymorphism
Citation: Delpire B, Van Loon E and Naesens M (2022) The Role of Fc Gamma Receptors in Antibody-Mediated Rejection of Kidney Transplants. Transpl Int 35:10465. doi: 10.3389/ti.2022.10465
Received: 25 February 2022; Accepted: 08 June 2022;
Published: 20 July 2022.
Copyright © 2022 Delpire, Van Loon and Naesens. This is an open-access article distributed under the terms of the Creative Commons Attribution License (CC BY). The use, distribution or reproduction in other forums is permitted, provided the original author(s) and the copyright owner(s) are credited and that the original publication in this journal is cited, in accordance with accepted academic practice. No use, distribution or reproduction is permitted which does not comply with these terms.
*Correspondence: Maarten Naesens, bWFhcnRlbi5uYWVzZW5zQGt1bGV1dmVuLmJl