- 1Department of Anesthesiology, Erasmus University Medical Center, Erasmus University Rotterdam, Rotterdam, Netherlands
- 2Department of Surgery, Division of Hepatopancreatobiliary Surgery and Liver Transplantation, University Medical Center Groningen, University of Groningen, Groningen, Netherlands
- 3Top Sector Life Sciences and Health (Health∼Holland), The Hague, Netherlands
- 4Department of Gastroenterology and Hepatology, University Medical Center Groningen, University of Groningen, Groningen, Netherlands
Prehabilitation improves surgical outcomes in patients undergoing surgery. However, patients preparing for orthotopic liver transplantation (OLT) are physically “frail” and suffer from comorbidities that generally hamper physical activity. This systematic review aims to evaluate the physical effects, safety and feasibility of prehabilitation in OLT candidates. Relevant articles were searched, in Embase, Web of Science, Cochrane, Medline and Google Scholar, to December 2021. Studies reporting on specified preoperative exercise programs, including adult OLT candidates with end-stage liver disease, with a model for end-stage liver disease (MELD) score ≥12 or Child-Pugh classification B/C, were included. This resulted in 563 potentially eligible studies, out of which eight were selected for inclusion, consisting of 1,094 patients (male sex 68%; mean age 51–61 years; mean MELD score 12-21). Six of the included studies were classified as low-quality by the GRADE system, and three studies had high risk for ineffectiveness of the training program according to the i-CONTENT tool. Significant improvement was observed in VO2 peak, 6-minute walking distance, hand grip strength, liver frailty index and quality of life. Feasibility ranged from an adherence of 38%–90% in unsupervised-to >94% in supervised programs. No serious adverse events were reported. In conclusion, prehabilitation in patients awaiting OLT appears to improve aerobic capacity, and seems feasible and safe. However, larger clinical trials are required to accurately examine the preoperative and postoperative effects of prehabilitation in this specific patient population.
Introduction
Poor physical fitness and functional status compromise postoperative functional recovery and lead to adverse postoperative outcomes, including complications, prolonged length of in-hospital stay, and mortality (1).
In current practice, patients who undergo (major) abdominal surgery are postoperatively supported by physical therapists and dieticians as part of the Enhanced Recovery After Surgery (ERAS®) program to accelerate postoperative recovery by enhancing perioperative health and reducing the impact of hospitalization and surgical stress (2,3). In addition, preoperative physical fitness measured by cardiopulmonary exercise tests has shown to be an independent predictor for postoperative morbidity and mortality after major abdominal surgery (4). Therefore, in the recent years, an increasing amount of scientific evidence focusses on preoperative “rehabilitation,” known as prehabilitation (5,6). Prehabilitation is aimed at strengthening the “psychophysiological reserve” and mitigating the postoperative surgical stress response to improve postoperative outcomes by enhancing preoperative general health and reducing individual risk factors (6).
Previous studies showed that prehabilitation programs are feasible, safe, and effective in patients scheduled for major abdominal surgery (7-9). However, the evidence regarding the beneficial effects of prehabilitation in patients awaiting orthotopic liver transplantation (OLT), a generally physically ‘frail’ patient population, is limited. OLT candidates not only exhibit key premorbid components of frailty, such as diminished functional capacity, sarcopenia, and decreased aerobic capacity, but may also suffer from cirrhosis-induced complications, such as ascites, hepatic encephalopathy, or variceal bleeding (10,11), which raises questions concerning their trainability. However, the waiting period for this procedure, on average 28 weeks in the Netherlands, 13–17 weeks in the United Kingdom, and 24 weeks in the United States of America, might be used to optimize physical condition by training prior to OLT (12-14).
Moreover, previous research in OLT candidates predicted a higher survival after OLT in patients with a higher anaerobic threshold (a submaximal exercise parameter of cardiorespiratory reserve) (15). Therefore, prehabilitation could possibly benefit patients in reducing morbidity and mortality during the waiting period or after OLT.
The primary objective of this systematic review is to evaluate the observed effects of preoperative training on physical and functional capacity, and to evaluate the effect of prehabilitation on postoperative surgical outcomes after OLT. The secondary objective is to determine the feasibility and safety of prehabilitation programs in patients awaiting OLT. In addition to the primary and secondary objectives, we aim to provide an overview of the studied prehabilitation programs, including their content and potential for success (16,17).
Materials and Methods
Study Design
This systematic review was conducted and reported in accordance with the Preferred Reporting Items for Systematic Reviews and Meta-Analyses (PRISMA) Statement (18,19), see Online Supplementary S1. Two authors (WJ, RH) independently reviewed the selected studies in EndNote X9© (Clarivate Analytics, Boston, MA, United States). Identified articles were screened on title, abstract, and, subsequently, on full-text. Disagreements during the selection process were discussed by the two reviewing authors (WJ and RH) and a third author (RJ) until consensus was reached.
Search Strategy
The search strategy was developed in collaboration with a clinical librarian and information specialist and was executed in Embase, Web of Science, Cochrane, Medline (PubMed) and Google Scholar. Free text words and MeSH terms related to prehabilitation and liver transplantation were used. Reference lists of relevant review articles and current treatment guidelines were screened for additional eligible articles. All studies published before 21 December 2021 were included for screening by title and abstract. The full literature database search strategy is described in Online Supplementary S2.
Eligibility Criteria
All peer-reviewed randomized, controlled, and cohort studies reporting a specified preoperative exercise program for adult (age ≥18 years) patients actively listed for OLT or with end-stage liver disease (ESLD). To assess ESLD, the model for end-stage liver disease (MELD) score, a disease severity scoring system used to improve organ allocation for patients on the liver transplantation waiting list, and the Child-Pugh classification were used. Studies that assessed patients with a laboratory or exception MELD score ≥12 or a Child-Pugh classification B or C were included. Animal studies, case-reports, systematic reviews, conference abstracts, duplicates and studies containing paediatric patients were excluded.
Quality Assessment
Quality assessment of included studies was executed by using the principles of the Grading of Recommendation, Assessment, Development, and Evaluation (GRADE) (20,21). For a transparent assessment of the potential effectiveness of the exercise therapy programs studied in trials, intervention programs were evaluated according to the international Consensus on Therapeutic Exercise Training (i-CONTENT) tool (17). The i-CONTENT is used to assess the therapeutic quality of exercise programs employed in clinical trials (17).
Primary and Secondary Outcomes
The primary outcome was defined as the observed effects of preoperative training programs on physical and functional capacity and surgical outcome. Physical and functional capacity was assessed by comparing outcomes such as pre- and post-training oxygen consumption at peak exercise (VO2-peak), 6-minute walking distance (6MWD), hand grip strength, and quality of life (QoL). Surgical outcome was assessed by comparing data on post-OLT complications, length of in-hospital stay, length of intensive care unit (ICU) stay, and mortality.
Secondary outcomes were safety and feasibility of study- and training programs. The safety of training programs was assessed by comparing the occurrence and types of serious adverse outcomes during the training. The feasibility of studies was assessed by comparing patients identified as eligible for inclusion with the total number of included patients. The feasibility of training programs was assessed by an evaluation of the adherence to the training programs during the waiting period prior to OLT.
Data Collection and Definitions
Following the screening and selection of included studies, data was extracted by two independent authors (WJ, RH). Patient characteristics extracted included age; sex; body mass index (BMI); (lab and/or exception) MELD score; Child-Pugh classification and comorbidities, including diabetes mellitus, cardiac disease, pulmonary disease, ascites, gastroesophageal varices, and hepatic encephalopathy. Data regarding primary and secondary outcomes were extracted and tabulated. In addition, rationales, designs of the training programs, data on duration, frequency of training and exercises, training intensity and context, supervision of the training programs, and their potential for success were tabulated to provide a detailed overview of the prehabilitation programs. Normally distributed variables are presented as means with standard deviation (SD) and skewed variables as medians with interquartile range (IQR).
Results
Search Results
The search of aforementioned databases provided a total of 892 articles possible for inclusion. After removing duplicates, 563 articles remained for screening by title and abstract. Of these, 510 were excluded based on titles and abstracts. The full-texts of the remaining 53 articles were assessed for eligibility and reviewed in detail, whereafter 47 papers were excluded and six papers were included (Figure 1). Eventually, another 12 potentially relevant articles were found by screening references from articles that were already included for analysis. Of this total of 18 remaining articles, another 10 were excluded (Figure 1), and eight full-text studies (11,22-28) remained for systematic analysis (Table 1).
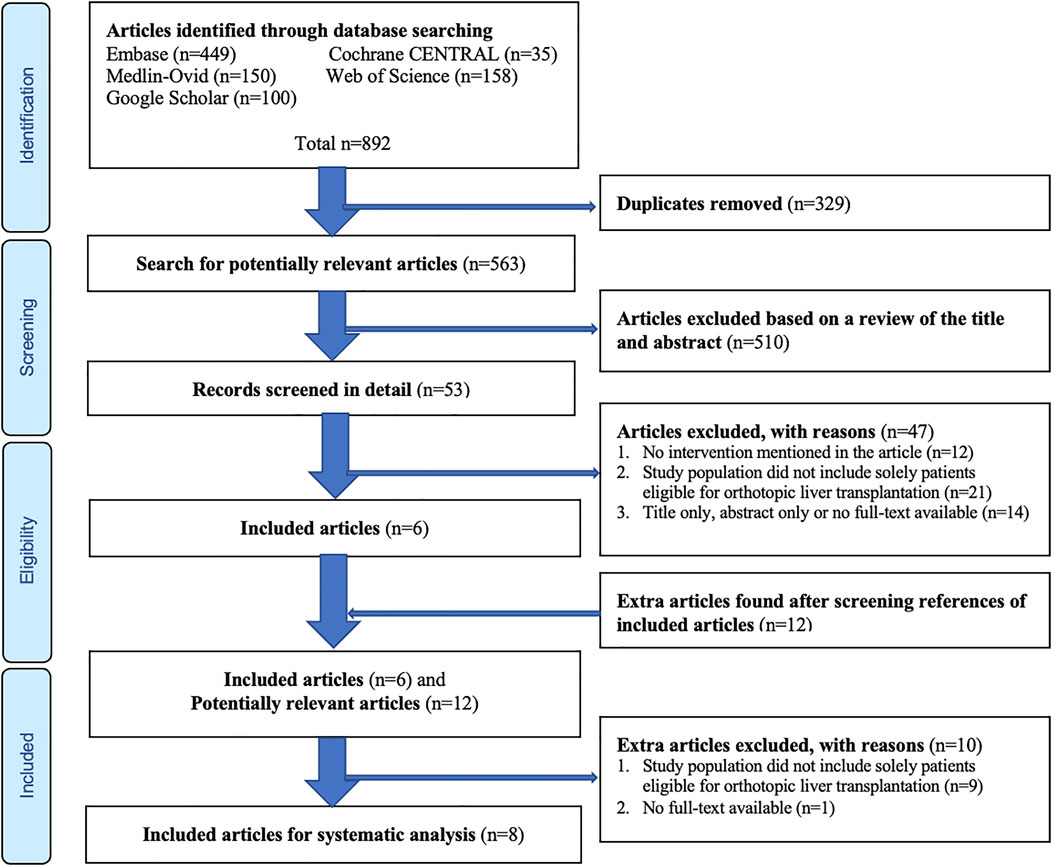
FIGURE 1. Flow diagram of the article selection procedure based on the Preferred Reporting Items for Systematic Reviews and Meta-Analyses (PRISMA) guideline.
Methodological Quality of Evidence Assessment
According to the GRADE system (20), two studies (24,28) were classified as moderate, while six (11,22-27) were classified as low-quality evidence, mainly due to the risk of bias and imprecision (Table 1). According to the i-CONTENT tool (17), five studies (23-28) were classified as low risk and three (11,22,27) as high risk for ineffectiveness of the training program. The main reason for high risk of ineffectiveness was due to unsupervised training (22,25,27,28) and missing reports on exercise-related adverse events (11,22,27,28) and adherence to the exercise program (11,22,23,27) (Table 2). For a detailed description of the grading process with the GRADE system and i-CONTENT tool, see Online Supplementary S3, S4, respectively.
Included Studies
Eight studies investigating a total of 1,094 patients (median (IQR): 20 (17–139)) were included. A total of three randomized controlled trials (RCTs) (22,24,27), one ambispective cohort study (28), three prospective cohort studies (23,25,26), and one retrospective cohort study (11) were included. The contexts of the training programs varied between supervised in-hospital training (11,23,24,26) and unsupervised home-based training (11,22,24,25,27,28).
Demographics, Primary and Secondary Outcomes
The majority of patients were male (68%). The mean or median age of the patients included in the training programs and control groups ranged from 51 to 61 and 54 to 56, respectively. In the studies reporting BMI, mean BMI in the training groups was ranging from 25.4 to 31 (22,25-28), which was higher than in the control groups (range 27–29) (22,26,27). The mean and median MELD-scores differed between studies, with five studies reporting mean or median MELD-scores between 12 and 14 in the training group (23-26,28), while, in the other three studies, these scores were above 16 in both the intervention and control groups (11,22,27). Six studies reported on the presence of one or more cirrhosis-induced comorbidities as ascites, gastroesophageal varices and hepatic encephalopathy (22-25,27,28). The number of patients with ascites ranged from 15% to 78% in the training groups (22-25,27,28) compared to 67%–75% in the control groups (22,27). The reported prevalence of hepatic encephalopathy ranged from 33% to 100% in the training groups (25,27,28) and 100% in the control groups (27). Three studies reported a prevalence of gastro-oesophageal varices ranging from 56% to 81% in the training group (24,27,28) and 88% in the control group (27). Baseline study characteristics and demographics are displayed in Table 1.
The primary outcomes reported on in the included studies varied and included alterations in spirometry results (22), alterations in frailty metrics (28), readmissions within 90 days post-OLT (11), and the safety and feasibility of training (23-25). The secondary outcomes were more uniform between the studies and included general QoL assessments (22-24,27), aerobic functioning after training (23-28), and adverse events during the program (11,22-27).
Intervention
Three of six studies that implemented unsupervised home-based training programs provided once-to-thrice weekly telephone contact for supervision or motivational support (11,25,27). The duration of training programs varied from six (26) to eight (24) to 12 weeks (22,23,25,27) and until OLT (28). The frequency of training varied per study; Limongi et al. provided a manual for daily, non-supervised, home-based exercise training (22), while Debette-Gratien et al. implemented twice-weekly, supervised, in-hospital gym training (23). Thrice weekly supervised in-hospital training was utilized by Wallen, Morkane, and Al-Judaibi et al. (11,24,26), Williams, Chen and Lin et al. advised non-supervised training up to five times per week, dependent on pre-defined weekly targets (25,27,28).
Physical training programs mainly consisted of aerobic training by cycle ergometer or walking programs (11,22-28), and strength exercises (11,22-25,28), or coughing and breathing exercises (22). Except for the interval training described by Morkane et al., Debette-Gratien et al. and Williams’ set goal to archive a work rate of 12–14 on the Borg scale of rate of perceived exertion (RPE-score) (23,25,26,29), no insight was provided into the intensity of the training programs in the other included studies. Al-Judaibi and Lin et al. provided education related to physical activity and dietary support in the training group (11,28), whereas, in Chen et al.’s study, both the intervention and control groups were provided with extra information regarding nutrition (27). Detailed information regarding the designs of the training programs, exclusion criteria, supervision, duration, and the risk of ineffectiveness is provided in Table 2.
Data-Analysis
Effect of Training on Physical Capacity
All the studies examining the physical effects of aerobic training reported some significant improvement in aerobic capacity (23-28) (Table 3). Debette-Gratien et al. reported a significant improvement in VO2 peak after training, from a mean VO2 peak of 21.5 (5.9) ml/kg/min before training to a mean VO2 peak value of 23.2 (5.9) ml/kg/min after training (p = 0.008) (23). In addition, Morkane et al. reported a significant VO2 peak improvement of 2.3 ml/kg/min in the training group (p = 0.02), while a decrease of 1.9 ml/kg/min was observed in the control group (p = 0.03) (26). Although Chen et al. found no significant improvement in VO2 peak after training (18 (7) before versus 17 (6) ml/kg/min after training, p = 0.42), they observed a decrease of 3 ml/kg/min (p = 0.08) in the control group (27). Debette-Gratien, Wallen, and Chen et al. reported significant improvements in walking distance after training (+40 m, p = 0.02; +16 m, p = 0.02 and +59m, p = 0.05, respectively) (23,24,27), while Lin et al. did not report a significant improvement in walking distance after training (F = 2.64, p = 0.07) (28). Furthermore, Debette-Gratien and Morkane et al. reported a significant improvement in grip strength (+7 kg, p = 0.008 and +3 kg, p = 0.05, respectively) after 12 weeks of training (23,26). However, in the study of Wallen et al., there was no significant improvement in grip strength after training (+0.4 kg, p = 0.24) (24). Regarding 6MWD and hand grip strength, no significant improvement or decline was observed in the control groups. Although Williams et al. did not report on VO2 peak or 6MWD, they did observe a significant improvement in aerobic capacity, measured by the incremental shuttle walk test (ISWT) (260 (70–1020) meter to 470 (190–880) meter, p < 0.01), and functional capacity, measured by the Short Physical Performance Battery Test (SPPBT) (9.5 (6–12) to 11.5 (9–12), p = 0.02), after 12 weeks of training. Lin et al. found a significant improvement of the liver frailty index (LFI) for all patients after training (F = 3.45, p = 0.01), and found an even larger effect in patients who adhered to >80% of the workout sessions until OLT (F = 8.10; p < 0.001) (28). Thereby, Lin et al. found a significant correlation with an improvement of the LFI and a survival advantages among included patients (28).
Perceived Health-Related Quality of Life Before and After Training
Four studies examined QoL before and after the training program while awaiting OLT (Table 4) (23-25,27). Williams et al. found an increase of 18% reported in the EuroQol visual analogue scale (EQ-VAS) questionnaire (p = 0.04) (25,30). And, although Williams et al. found no differences in median hospital anxiety and depression score (HADS) (10 (1–26) before training versus 7 (0–22) after training, p = 0.13), an increase of proportion of patients reporting no problems with mobility (44%) and pain/discomfort (56%) in the EuroQol 5-Dimension 5-Level (EQ-5D-5L) instrument was found after 12 weeks of prehabilitation (25). Debette-Gratien, Wallen, and Chen et al. found no differences in QoL between the training and control groups or between pre- and post-training on the SF-36 (24) or the HR-QoL (23,24,27). However, in Chen et al.’s study, an improvement was observed on the sickness impact profile (SIP) in the training group (−4.2, p = 0.10), while the SIP in the control group worsened (+4.2, p = 0.07) (27).
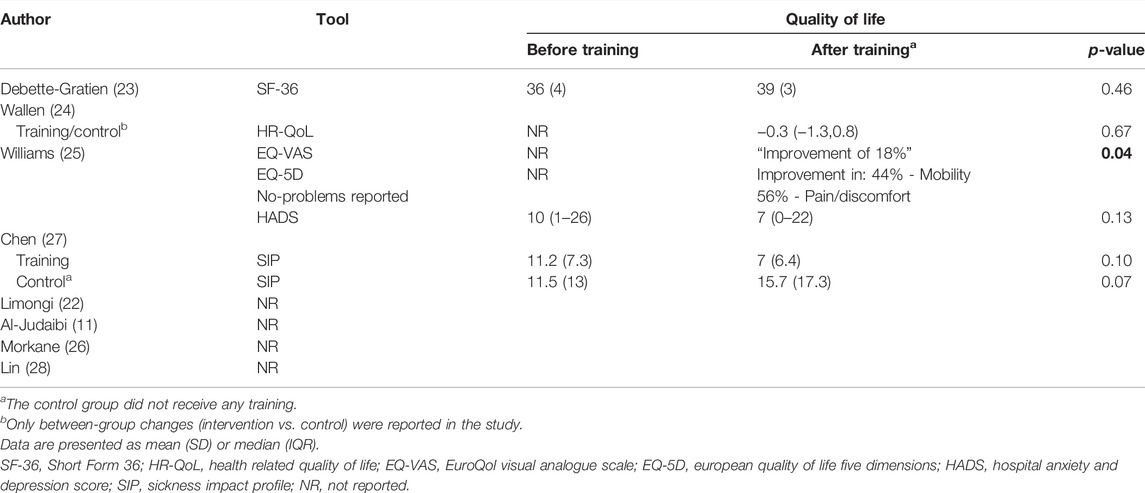
TABLE 4. Effect of training on quality of life in patients awaiting orthotopic liver transplantation.
Effects of Training on Length of Hospital Stay After OLT
Two studies (11,26) described differences in the length of in-hospital stay after OLT between the training groups and control groups (Table 5). Al-Judaibi et al. found a significantly shorter median length of ICU stay before transplantation in the intervention group compared to the control group (n = 458, 2 vs. 3 days, p = 0.01), however, no significant difference was observed in the length of in-hospital stay after OLT (11). Morkane et al. found no difference in the median length of ICU stay between the intervention and control groups (2 (4) versus 4 (5.5), p = 0.77), but found a significant difference in postoperative median length of hospital stay between the training group and control group (13 (7–19) versus 30 (17–43), p = 0.02) (26).
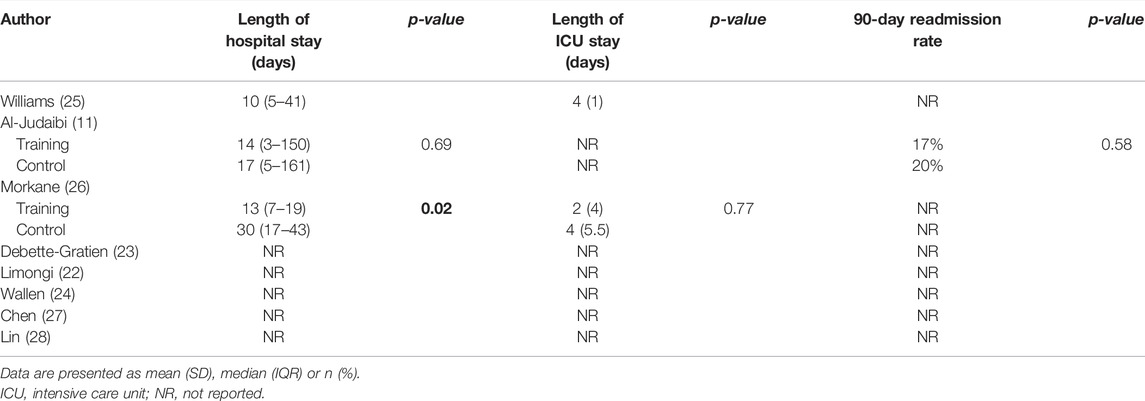
TABLE 5. Effect of training on postoperative surgical outcome after orthotopic liver transplantation.
Feasibility of the Studies Performed
Three studies reported on the participants identified for possible inclusion and the reasons for exclusion. Wallen et al. identified 38 patients, of whom 15 declined to participate; one patient was transplanted before the start of the training program, and another was delisted before commencement, leaving 21 (55%) suitable for inclusion (24). Chen et al. identified 227 OLT candidates and excluded 210 (93%) for various reasons: 85 because of the presence of a hepatocellular carcinoma, 73 due to logistic or transport issues, 35 because of cardiopulmonary or metabolic diseases, 14 because of being delisted as OLT candidates, two due to repeated hospitalization, and one because that patient already walked more than 10,000 steps per day (27). Williams et al. randomly selected 46 patients from the OLT waiting list: 32 (70%) were eligible for study entry, with patients awaiting a re-transplantation being the most common reason for exclusion (5 out of 46; 11%). Of the 32 patients deemed eligible, six (18.8%) declined participation and eight (25%) underwent OLT prior to study visit one. Therefore, a total 18 out of 32 eligible patients (56.2%) were enrolled in the study (25). Al-Judaibi et al. Debette-Gratien et al. and Lin et al. included consecutive patients and had a study feasibility of 100% (11,23,28). Limongi et al. identified 42 patients and included 17 (40%) in their study without listing reasons for exclusion (22), and Morkane et al. did not report on patients eligible for inclusion (26). No studies excluded patients with gastro-oesophageal varices treated with β-blockers (Table 2).
Feasibility and Safety of Training Programs
Outcomes regarding safety, feasibility, and adherence to the training programs are displayed in Table 6. Three author groups reported the feasibility and safety of their training programs as their primary outcome (24-26). Williams et al. defined feasibility as the absence of training-related serious adverse events; the eligibility of 66% or more of patients who are actively listed on the OLT waiting list; and more than 66% adherence to the daily step count and resistance exercises and completion of 6 weeks training (25). In their study, 82% of the patients adhered to daily step targets and 90% to the twice-weekly exercises (25). Morkane et al. reported a 94% adherence with all exercises (26), and Wallen et al. reported a 95% and 75% adherence to supervised and unsupervised exercise training, respectively (24). Lin et al. reported an adherence to minimally one follow-up physical therapy session of 69% (28). Patients’ self-reported adherence varied from adherence of 4–5 days/week in 38% of the patients, to 1–3 days/week in 51% of the patients and 0 days/week in 11% of the patients (28).
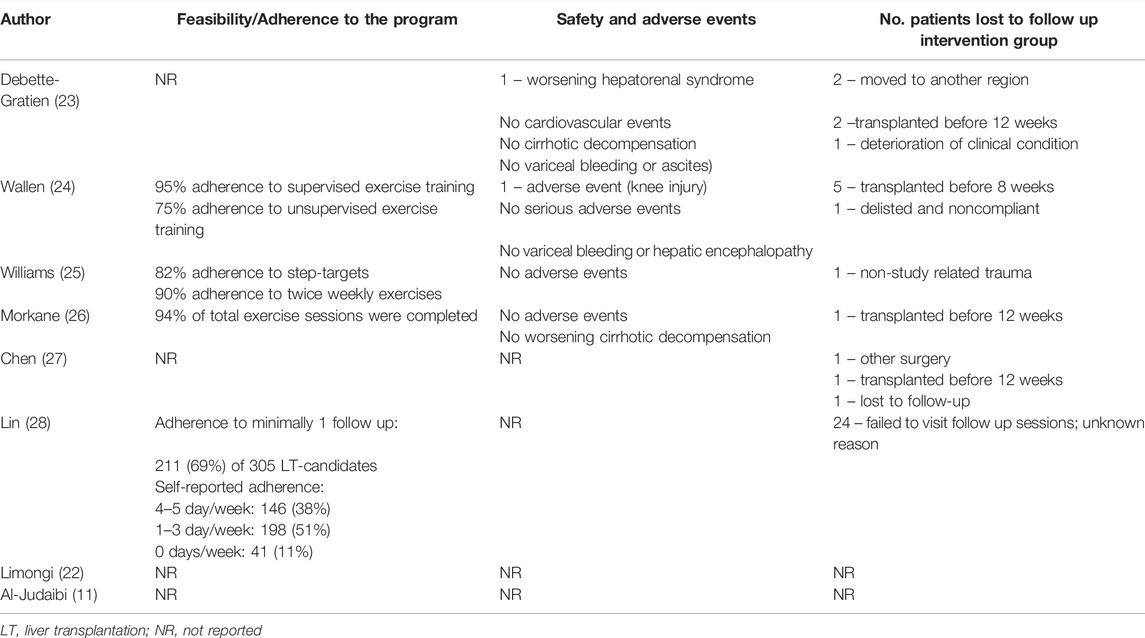
TABLE 6. Feasibility and safety of prehabilitation in patients awaiting orthotopic liver transplantation.
Four studies (23-26) described the potential of serious adverse events resembling cardiovascular events, cirrhosis decompensation, variceal bleeding or hepatic encephalopathy, but none of the authors reported any of these events occurring during the study. Wallen et al. reported on one adverse event (knee injury, one out of 11 patients (9.1%)) that occurred during training (24). In the study of Debette-Gratien et al., one patient (one out of 13 patients (7.7%)) stopped training due to worsening of their hepatorenal syndrome (23). Most common reason for dropping out of the program was because of transplantation before the end of the study period. All reasons why patients were lost to follow-up are listed in Table 6.
Discussion
The aim of this systematic review was to evaluate the effect of prehabilitation on physical capacity and surgical outcome in patients actively waiting for OLT. Six out of eight studies demonstrated significant improvements in aerobic or physical capacity (23-28). Adherence to the training programs was 69% or higher, and none of the included studies reported any serious adverse events. Therefore, these findings imply that prehabilitation programs are safe, feasible, and, potentially, effective for OLT-candidates.
In the past, one other review and one meta-analysis have been conducted in patients with chronic liver disease to assess the effect of training on their physical capacity (31,32). And although this current review shows resemblance to these previously conducted reviews, the majority of their included studies excluded potential OLT candidates and patients with MELD score ≥12, while this current review solely focussed on patients with ESLD awaiting OLT (31,32). For example, in the review conducted by Williams et al., the authors concluded that moderate‐to-high intensity exercise can improve the physical components of frailty and QoL in patients with chronic liver disease, but that it remained to be elucidated whether this also applies to patients with Child Pugh B/C decompensated cirrhosis (33-39). In the review of Brustia et al., where not solely patients awaiting OLT were included, no adverse events were caused by the training, but neither an improvement in physical capacity was observed (32).
When elaborating on the physical effects of prehabilitation in OLT candidates, previous literature has shown that preoperative VO2 peak and MELD score are independent prognostic factors of mortality and duration of hospitalization during both the pre- and post-transplantation periods (15,40-42). Hence, it can be hypothesized that increased VO2 peak due to training, could improve surgical outcome for the OLT candidate. The ability to increase this physical capacity with training was shown by several studies included in this review (23-27). The studies of Debette-Gratien, Morkane and Williams et al. all found a significant improvement in aerobic capacity after training (23,25,26). Their results, however, differed from the study by Chen et al., who found no difference in VO2 peak after training (27). This difference in results might be explained by the differences in design of the training programs of the three studies: Debette-Gratien and Morkane et al. provided specified supervised aerobic training with a cycle ergometer, Williams et al. used video guided exercises and non-supervised walking training, and Chen et al. solely implemented non-supervised walking training (23,25-27). Thereby, only three out of eight studies outlined the aerobic intensity of the exercises (23,25,26). Debette-Gratien et al. and Morkane et al. based their patient-adjusted aerobic training protocol on VO2 peak and on the anaerobic threshold which was objectified by CPET (23,26). Williams et al. used a subjective scale where patients were asked to achieve a work rate of 12–14 on the Borg scale (25). To speculate, these results should be interpreted with caution, but suggest that supervised aerobic cycling training by use of a patient-adjusted protocol could be more beneficial than unsupervised walking training. The hypothesis that physical training improves postoperative recovery was only described in three out of the eight included studies (11,25,26), and seems to be consistent with the findings of Lin et al., who found a significant correlation between survival advantage with improvement of the LFI score (28), and Morkane et al., who found a significant median difference of 17 days in the length of in-hospital stay between the intervention and control groups (26). However, in contrast with Morkane et al.’s finding, Al-Judaibi et al. found no difference in the length of in-hospital stay or 90-day readmission rate (11). The differences between the studies of Morkane and Al-Judaibi et al. may be explained by the studies’ population sizes (n = 17 vs. n = 458, respectively) and the significantly older population with more comorbidities in the training group compared to the control group in the study of Al-Judaibi et al. (11), while in the study of Morkane et al. no significant differences in baseline demographics of the two groups were reported (26).
Debette-Gratien et al. were able to include 100% of eligible candidates in their study (23), while Chen et al. only included 7% of eligible candidates. This discrepancy between eligible and eventually included patients could be caused by tight inclusion criteria, but results in a questionable feasibility of the study and could increase the risk of potential attrition bias. However, when evaluating feasibility of the training programs, all studies that mentioned adherence to the program reported a 38–90% adherence in unsupervised exercise training (24,25,28) and 94% or higher adherence to supervised exercise training (24,26), suggesting a high feasibility of prehabilitation programs in OLT candidates. These findings are somewhat surprising since the psychological burden on the OLT candidate is high (43), and the long waiting time and presence of symptoms related to liver cirrhosis possibly corrode compliance and motivation (34). Nonetheless, the dropout rate was low in all studies, and the most common reason for dropout was because patients were transplanted before the end of the study period.
This review has several limitations. First of all, this review was not pre-registered on the PROSPERO database, which could have caused reduced transparency of the applied search strategy of this review. Secondly, there are certain limitations regarding the studied evidence: most included studies consisted of small patient populations, and focused on different primary outcomes, which made the comparison and analysis of the studies challenging. In addition, most of the studies were non-randomized, which leads to a reduction in the analysis strength of this review. Finally, as the values of the baseline and post-training outcomes are not independent of each other, and correlations were not reported by the individual studies, meta-analyses were not possible (44). The high heterogeneity and lack of high-quality trials make it difficult to draw conclusions on the true effect of prehabilitation, when taking infrastructural differences, waiting time and clinical status as prognostic factors of success of the training programs in account. However, by strictly including only studies with patients having ESLD who are actively waitlisted for OLT, a bias of representing a “healthier” study group is prevented. Therefore, the strength of this review is, therefore, to represent the “most physically frail” patients, namely the OLT candidates with ESLD.
In our opinion, home-based training, which is supervised by a dedicated physical therapist and is combined with nutritional and educational support by a dietician, could be suitable for preoperative optimization until OLT. Patients might make some progress during these weeks of training, but, most importantly, deterioration of aerobic capacity could be prevented (27) and the number of hospital admissions due to decompensated liver disease during the waiting period could be reduced (45). To the best of our knowledge, the economic burden of the implementation of a prehabilitation program in this patient population has not been studied yet. One can imagine that the supervision and provision of a personalized training program for this frail population requires professional health-care workers as physiotherapists and dieticians. However, since previous studies showed cost-effectiveness for prehabilitation in patients undergoing abdominal surgery (46), we think that investing in personalized training programs for this specifically frail population could be beneficial. However, the effects of physical training in this patient population are still not decisive, and objectively measured effects of structured training programs on days of hospitalization, presence of complications and functional evolution after transplantation are scarce. Therefore, this review emphasizes the need for large (multicentre) longitudinal trials that not only study the physical effects, but also focus on possible improvement of surgical outcomes after a longer duration of training during the waiting period prior to OLT. Randomizing between training and no training is, in our opinion, not ethically justifiable, because various studies (45,47,48) have shown the benefits of improved physical capacity, activity, and muscle status with surgical outcome.
In conclusion, this systematic review found that prehabilitation in patients actively listed for OLT may improve aerobic and functional capacity, and, more importantly, that deterioration in aerobic and functional capacity could be countered by prehabilitation. Thereby, since no serious adverse events were reported and adherence to the training programs was high, we conclude that prehabilitation is safe and feasible in the OLT candidate. Thus, from our point of view, all patients awaiting OLT, especially the most physically frail ones, should be enrolled in predefined prehabilitation programs.
Data availability statement
The datasets used and/or analyzed during the current study are available from the corresponding author on reasonable request.
Author contributions
WJ and RH were involved in the review of the concept, literature search, design, manuscript preparation and review/editing of final manuscript. NM, FC, JK, and RJ were involved in the review of the concept and review/editing of final manuscript. All authors read and approved the final manuscript.
Conflict of Interest
The authors declare that the research was conducted in the absence of any commercial or financial relationships that could be construed as a potential conflict of interest.
Acknowledgments
The authors would like to thank Wichor M. Bramer, PhD, as a biomedical information specialist from the Erasmus University Medical Center medical library for the collaboration in developing and updating the literary search strategy. The authors would also like to thank Sanne E. Hoeks, PhD as a clinical epidemiologist from the Erasmus University Medical Center for assistance with the statistical analyses of the results.
Supplementary Material
The Supplementary Material for this article can be found online at: https://www.frontierspartnerships.org/articles/10.3389/ti.2022.10330/full#supplementary-material
Abbreviations
6MWD, six-minute walking distance; ERAS, enhanced recovery after surgery; ESLD, end-stage liver disease; F, delta point; GRADE, Grades of Recommendation, Assessment, Development and Evaluation for quality assessment of clinical studies; HR-QoL, health-related quality of life; i-CONTENT, international Consensus on Therapeutic Exercise aNd Training; ICU, intensive care unit; IQR, interquartile range; LFI, liver frailty index; MELD, model for end-stage liver disease; OLT, orthotopic liver transplantation; PRISMA, Preferred Reporting Items for Systematic Reviews and Meta-Analyses; PROSPERO, prospective register of systematic reviews; RCT, randomized controlled trial; SD, standard deviation; VO2 peak, oxygen consumption at peak exercise.
References
1. Dronkers, JJ, Chorus, AMJ, van Meeteren, NLU, and Hopman-Rock, M. The Association of Pre-operative Physical Fitness and Physical Activity with Outcome after Scheduled Major Abdominal Surgery. Anaesthesia (2013) 68(1):67–73. doi:10.1111/anae.12066
2. Mortensen, K, Nilsson, M, Slim, K, Schäfer, M, Mariette, C, Braga, M, et al. Consensus Guidelines for Enhanced Recovery after Gastrectomy: Enhanced Recovery after Surgery (ERAS®) Society Recommendations. Br J Surg (2014) 101(10):1209–29. doi:10.1002/bjs.9582
3. Lee, Y, Yu, J, Doumouras, AG, Li, J, and Hong, D. Enhanced Recovery after Surgery (ERAS) versus Standard Recovery for Elective Gastric Cancer Surgery: A Meta-Analysis of Randomized Controlled Trials. Surg Oncol (2020) 32:75–87. doi:10.1016/j.suronc.2019.11.004
4. Prentis, JM, Trenell, MI, Vasdev, N, French, R, Dines, G, Thorpe, A, et al. Impaired Cardiopulmonary reserve in an Elderly Population Is Related to Postoperative Morbidity and Length of Hospital Stay after Radical Cystectomy. BJU Int (2013) 112(2):E13–9. doi:10.1111/bju.12219
5. Bongers, BC, Dejong, CHC, and den Dulk, M. Enhanced Recovery after Surgery Programmes in Older Patients Undergoing Hepatopancreatobiliary Surgery: what Benefits Might Prehabilitation Have? Eur J Surg Oncol (2021) 47(3):551–9. doi:10.1016/j.ejso.2020.03.211
6. Durrand, J, Singh, SJ, and Danjoux, G. Prehabilitation.. Clin Med (2019) 19(6):458–64. doi:10.7861/clinmed.2019-0257
7. van Wijk, L, Berkel, AEM, Buis, CI, Bongers, BC, and Klaase, JM. Preoperative home-based Exercise Prehabilitation in Patients Scheduled for Liver or Pancreatic Resection: The First Results. HPB (Oxford) (2020) 46(2):S231. doi:10.1016/j.hpb.2020.04.086
8. van Wijk, L, van der Snee, L, Buis, CI, Hentzen, JEKR, Haveman, ME, and Klaase, JM. A Prospective Cohort Study Evaluating Screening and Assessment of Six Modifiable Risk Factors in HPB Cancer Patients and Compliance to Recommended Prehabilitation Interventions. Perioper Med (2021) 10(1):5. doi:10.1186/s13741-020-00175-z
9. Hughes, MJ, Hackney, RJ, Lamb, PJ, Wigmore, SJ, Christopher Deans, DA, and Skipworth, RJE. Prehabilitation before Major Abdominal Surgery: A Systematic Review and Meta-Analysis. World J Surg (2019) 43(7):1661–8. doi:10.1007/s00268-019-04950-y
10. Beyer, N, Aadahl, M, Strange, B, Kirkegaard, P, Hansen, BA, Mohr, T, et al. Improved Physical Performance after Orthotopic Liver Transplantation. Liver Transpl Surg (1999) 5(4):301–9. doi:10.1002/lt.500050406
11. Al-Judaibi, B, Alqalami, I, Sey, M, Qumosani, K, Howes, N, Sinclair, L, et al. Exercise Training for Liver Transplant Candidates. Transpl Proc (2019) 51(10):3330–7. doi:10.1016/j.transproceed.2019.08.045
12. Tieleman, M, van den Berg, AP, Hoek, B, Polak, WG, Dubbeld, J, Porte, RJ, et al. Komt mijn nieuwe lever wel op tijd?” Overlevingskansen van patiënten op de wachtlijst voor levertransplantatie. Ned Tijdschr Geneeskd (2018) 162:D2159.
13.National Health Services (NHS). Blood and Transplant. Liver Transplantation Data [Internet]. [cited 2022 Jun 17] (2020). Available from: https://www.nhsbt.nhs.uk/organ-transplantation/liver/receiving-a-liver/how-long-is-the-wait-for-a-liver/ (Accessed June 15, 2022).
14.U.S. Department of Health and Human Services (HHS). OPTN/SRTR 2019 Annual Data Report: Liver [Internet]. [cited 2022 Jun 17] (2019). Available from: https://srtr.transplant.hrsa.gov/annual_reports/2019/Liver.aspx (Accessed June 15, 2022).
15. Prentis, JM, Manas, DMD, Trenell, MI, Hudson, M, Jones, DJ, and Snowden, CP. Submaximal Cardiopulmonary Exercise Testing Predicts 90-day Survival after Liver Transplantation. Liver Transpl (2012) 18(2):152–9. doi:10.1002/lt.22426
16. Hoogeboom, TJ, Dronkers, JJ, Hulzebos, EHJ, and van Meeteren, NLU. Merits of Exercise Therapy before and after Major Surgery. Curr Opin Anaesthesiol (2014) 27(2):161–6. doi:10.1097/ACO.0000000000000062
17. Hoogeboom, TJ, Kousemaker, MC, van Meeteren, NL, Howe, T, Bo, K, Tugwell, P, et al. I-CONTENT Tool for Assessing Therapeutic Quality of Exercise Programs Employed in Randomised Clinical Trials. Br J Sports Med (2020) 55:1153–60. doi:10.1136/bjsports-2019–10163010.1136/bjsports-2019-101630
18. Moher, D, Shamseer, L, Clarke, M, Ghersi, D, Liberati, A, Petticrew, M, et al. Preferred Reporting Items for Systematic Review and Meta-Analysis Protocols (PRISMA-P) 2015 Statement. Syst Rev (2015) 4(1):1. doi:10.1186/2046-4053-4-1
19. Liberati, A, Altman, DG, Tetzlaff, J, Mulrow, C, Gøtzsche, PC, Ioannidis, JPA, et al. The PRISMA Statement for Reporting Systematic Reviews and Meta-Analyses of Studies that Evaluate Health Care Interventions: Explanation and Elaboration. Plos Med (2009) 6(7):e1000100. doi:10.1371/journal.pmed.1000100
20. Guyatt, GH, Oxman, AD, Vist, GE, Kunz, R, Falck-Ytter, Y, Alonso-Coello, P, et al. GRADE: an Emerging Consensus on Rating Quality of Evidence and Strength of Recommendations. BMJ (2008) 336(7650):924–6. doi:10.1136/bmj.39489.470347.AD
21. Guyatt, GH, Oxman, AD, Sultan, S, Glasziou, P, Akl, EA, Alonso-Coello, P, et al. GRADE Guidelines: 9. Rating up the Quality of Evidence. J Clin Epidemiol (2011) 64(12):1311–6. doi:10.1016/j.jclinepi.2011.06.004
22. Limongi, V, Dos Santos, DC, Da Silva, AMO, Ataide, EC, Mei, MFT, Udo, EY, et al. Effects of a Respiratory Physiotherapeutic Program in Liver Transplantation Candidates. Transpl Proc (2014) 46(6):1775–7. doi:10.1016/j.transproceed.2014.05.044
23. Debette-Gratien, M, Tabouret, T, Antonini, MT, Dalmay, F, Carrier, P, Legros, R, et al. Personalized Adapted Physical Activity before Liver Transplantation: Acceptability and Results. Transplantation (2015) 99(1):145–50. doi:10.1097/TP.0000000000000245
24. Wallen, MP, Keating, SE, Hall, A, Hickman, IJ, Pavey, TG, Woodward, AJ, et al. Exercise Training Is Safe and Feasible in Patients Awaiting Liver Transplantation: A Pilot Randomized Controlled Trial. In: Liver Transplantation, 25. John Wiley & Sons (2019). p. 1576–80. doi:10.1002/lt.25616
25. Williams, FR, Vallance, A, Faulkner, T, Towey, J, Durman, S, Kyte, D, et al. Home-based Exercise in Patients Awaiting Liver Transplantation: A Feasibility Study.. Liver Transpl (2019) 25(7):995–1006. doi:10.1002/lt.25442
26. Morkane, CM, Kearney, O, Bruce, DA, Melikian, CN, and Martin, DS. An Outpatient Hospital-Based Exercise Training Program for Patients with Cirrhotic Liver Disease Awaiting Transplantation: A Feasibility Trial. Transplantation (2019) 104(1):97–103. doi:10.1097/TP.0000000000002803
27. Chen, HW, Ferrando, A, White, MG, Dennis, RA, Xie, J, Pauly, M, et al. Home-Based Physical Activity and Diet Intervention to Improve Physical Function in Advanced Liver Disease: A Randomized Pilot Trial. Dig Dis Sci (2020) 65(11):3350–9. doi:10.1007/s10620-019-06034-2
28. Lin, FP, Visina, JM, Bloomer, PM, Dunn, MA, Josbeno, DA, Zhang, X, et al. Prehabilitation-driven Changes in Frailty Metrics Predict Mortality in Patients with Advanced Liver Disease. Am J Gastroenterol (2021) 116(10):2105–17. doi:10.14309/ajg.0000000000001376
29. Borg, GA. Psychophysical Bases of Perceived Exertion. Med Sci Sports Exerc (1982) 14(5):377–81. doi:10.1249/00005768-198205000-00012
30. Janssen, MF, Pickard, AS, Golicki, D, Gudex, C, Niewada, M, Scalone, L, et al. Measurement Properties of the EQ-5D-5L Compared to the EQ-5D-3L across Eight Patient Groups: a Multi-Country Study. Qual Life Res (2013) 22(7):1717–27. doi:10.1007/s11136-012-0322-4
31. Williams, FR, Berzigotti, A, Lord, JM, Lai, JC, and Armstrong, MJ. Review Article: Impact of Exercise on Physical Frailty in Patients with Chronic Liver Disease. Aliment Pharmacol Ther (2019) 50(9):988–1000. doi:10.1111/apt.15491
32. Brustia, R, Savier, E, and Scatton, O. Physical Exercise in Cirrhotic Patients: Towards Prehabilitation on Waiting List for Liver Transplantation. A Systematic Review and Meta-Analysis. Clin Res Hepatol Gastroenterol (2018) 42(3):205–15. doi:10.1016/j.clinre.2017.09.005
33. Berzigotti, A, Albillos, A, Villanueva, C, Genescá, J, Ardevol, A, Augustín, S, et al. Effects of an Intensive Lifestyle Intervention Program on portal Hypertension in Patients with Cirrhosis and Obesity: The SportDiet Study. Hepatology (2017) 65(4):1293–305. doi:10.1002/hep.28992
34. Hallsworth, K, Jopson, L, Jones, DE, and Trenell, MI. Exercise Therapy in Primary Biliary Cirrhosis: the Importance of Moving while Sitting on a Surgical Waiting List-A Case Study. Frontline Gastroenterol (2016) 7(3):167–9. doi:10.1136/flgastro-2015-100672
35. Kruger, C, McNeely, ML, Bailey, RJ, Yavari, M, Abraldes, JG, Carbonneau, M, et al. Home Exercise Training Improves Exercise Capacity in Cirrhosis Patients: Role of Exercise Adherence. Sci Rep (2018) 8(1):99. doi:10.1038/s41598-017-18320-y
36. Nishida, Y, Ide, Y, Okada, M, Otsuka, T, Eguchi, Y, Ozaki, I, et al. Effects of home-based Exercise and Branched-Chain Amino Acid Supplementation on Aerobic Capacity and Glycemic Control in Patients with Cirrhosis. Hepatol Res (2017) 47(3):E193–200. doi:10.1111/hepr.12748
37. Román, E, García-Galcerán, C, Torrades, T, Herrera, S, Marín, A, Doñate, M, et al. Effects of an Exercise Programme on Functional Capacity, Body Composition and Risk of Falls in Patients with Cirrhosis: A Randomized Clinical Trial. PLoS One (2016) 11(3):e0151652. doi:10.1371/journal.pone.0151652
38. Román, E, Torrades, MT, Nadal, MJ, Cárdenas, G, Nieto, JC, Vidal, S, et al. Randomized Pilot Study: Effects of an Exercise Programme and Leucine Supplementation in Patients with Cirrhosis. Dig Dis Sci (2014) 59(8):1966–75. doi:10.1007/s10620-014-3086-6
39. Zenith, L, Meena, N, Ramadi, A, Yavari, M, Harvey, A, Carbonneau, M, et al. Eight Weeks of Exercise Training Increases Aerobic Capacity and Muscle Mass and Reduces Fatigue in Patients with Cirrhosis. Clin Gastroenterol Hepatol (2014) 12(11):1920–6. doi:10.1016/j.cgh.2014.04.016
40. Epstein, SK, Freeman, RB, Khayat, A, Unterborn, JN, Pratt, DS, and Kaplan, MM. Aerobic Capacity Is Associated with 100-day Outcome after Hepatic Transplantation. Liver Transpl (2004) 10(3):418–24. doi:10.1002/lt.20088
41. Trojetto, T, Elliott, RJ, Rashid, S, Wong, S, Dlugosz, K, Helm, D, et al. Availability, Characteristics, and Barriers of Rehabilitation Programs in Organ Transplant Populations across Canada. Clin Transpl (2011) 25(6):E571–8. doi:10.1111/j.1399-0012.2011.01501.x
42. Dharancy, S, Lemyze, M, Boleslawski, E, Neviere, R, Declerck, N, Canva, V, et al. Impact of Impaired Aerobic Capacity on Liver Transplant Candidates. Transplantation (2008) 86(8):1077–83. doi:10.1097/TP.0b013e318187758b
43. Yadav, A, Chang, YH, Carpenter, S, Silva, AC, Rakela, J, Aqel, BA, et al. Relationship between Sarcopenia, Six-Minute Walk Distance and Health-Related Quality of Life in Liver Transplant Candidates. Clin Transpl (2015) 29(2):134–41. doi:10.1111/ctr.12493
44. Cuijpers, P, Weitz, E, Cristea, IA, and Twisk, J. Pre-post Effect Sizes Should Be Avoided in Meta-Analyses. Epidemiol Psychiatr Sci (2017) 26(4):364–8. doi:10.1017/S2045796016000809
45. Coca-Martinez, M, Lopez-Hernandez, A, Montane-Muntane, M, Arguis, MJ, Gimeno-Santos, E, Navarro-Ripoll, R, et al. Multimodal Prehabilitation as Strategy for Reduction of Postoperative Complications after Cardiac Surgery: a Randomised Controlled Trial Protocol. BMJ Open (2020) 10(12):e039885. doi:10.1136/bmjopen-2020-039885
46. Dholakia, J, Cohn, DE, Straughn, JM, and Dilley, SE. Prehabilitation for Medically Frail Patients Undergoing Surgery for Epithelial Ovarian Cancer: a Cost-Effectiveness Analysis. J Gynecol Oncol (2021) 32(6):e92. doi:10.3802/jgo.2021.32.e92
47. Snowden, CP, Prentis, J, Jacques, B, Anderson, H, Manas, D, Jones, D, et al. Cardiorespiratory Fitness Predicts Mortality and Hospital Length of Stay after Major Elective Surgery in Older People. Ann Surg (2013) 257(6):999–1004. doi:10.1097/SLA.0b013e31828dbac2
Keywords: prehabilitation, orthotopic liver transplantation, physical exercise training, aerobic capacity, safety, feasibility
Citation: Jetten WD, Hogenbirk RNM, Van Meeteren NLU, Cuperus FJC, Klaase JM and De Jong R (2022) Physical Effects, Safety and Feasibility of Prehabilitation in Patients Awaiting Orthotopic Liver Transplantation, a Systematic Review. Transpl Int 35:10330. doi: 10.3389/ti.2022.10330
Received: 31 December 2021; Accepted: 24 August 2022;
Published: 08 September 2022.
Copyright © 2022 Jetten, Hogenbirk, Van Meeteren, Cuperus, Klaase and De Jong. This is an open-access article distributed under the terms of the Creative Commons Attribution License (CC BY). The use, distribution or reproduction in other forums is permitted, provided the original author(s) and the copyright owner(s) are credited and that the original publication in this journal is cited, in accordance with accepted academic practice. No use, distribution or reproduction is permitted which does not comply with these terms.
*Correspondence: Wesley D. Jetten, d2VzbGV5LmpldHRlbkBnbWFpbC5jb20=
†ORCID: Wesley D. Jetten, orcid.org/0000-0001-8896-6608; Rianne N. M. Hogenbirk, orcid.org/0000-0002-7723-0256; Nico L. U. Van Meeteren, orcid.org/0000-0002-3067-4167; Frans J. C. Cuperus, orcid.org/0000-0001-6742-1510; Joost M. Klaase, orcid.org/0000-0002-4409-2280; Renate De Jong, orcid.org/0000-0003-4847-0610
‡These authors have contributed equally to this work