Effects of botulinum neurotoxin on regularity of head oscillations in cervical dystonia
- 1National VA Parkinson Consortium Center, Louis Stokes Cleveland VA Medical Center, Cleveland, OH, United States
- 2Department of Neurology, Emory University, Atlanta, GA, United States
- 3Department of Neurology, Johns Hopkins University, Baltimore, MD, United States
- 4Department of Neurology, University Hospitals, Case Western Reserve University, Cleveland, OH, United States
- 5Department of Biomedical Engineering, Case Western Reserve University, Cleveland, OH, United States
Introduction: This study explores the effects of botulinum neurotoxin (BoNT) on the relationship between dystonia and tremor, specifically focusing on cervical dystonia (CD) and its connection to head tremor.
Methods: Fourteen CD patients were recruited; eight (57%) with clinically observable head oscillations were included in further analysis. A high-resolution magnetic search coil system precisely measured head movements, addressing two questions: 1) BoNT’s effects on head movement amplitude, frequency, and regularity, and 2) BoNT’s influence on the relationship between head position and head oscillations. For the first question, temporal head position measurements of three patients were analyzed before and after BoNT injection. The second question examined the effects of BoNT injections on the dependence of the oscillations on the position of the head.
Results: Three distinct trends were observed: shifts from regular to irregular oscillations, transitions from irregular to regular oscillations, and an absence of change. Poincaré analysis revealed that BoNT induced changes in regularity, aligning oscillations closer to a consistent “set point” of regularity. BoNT injections reduced head oscillation amplitude, particularly in head orientations linked to high-intensity pre-injection oscillations. Oscillation frequency decreased in most cases, and overall variance in the amplitude of head position decreased post-injection.
Discussion: These findings illuminate the complexity of CD but also suggest therapeutic potential for BoNT. They show that co-existing mechanisms contribute to regular and irregular head oscillations in CD, which involve proprioception and central structures like the cerebellum and basal ganglia. These insights advocate for personalized treatment to optimize outcomes that is based on individual head oscillation characteristics.
Introduction
The dystonias encompass a group of conditions marked by excessive muscle contractions leading to involuntary postures or movements characterized by repetitive or contorted postures [1–3]. As a collective entity, they stand as the third most prevalent movement disorder following tremors and Parkinson’s disease, impacting over 3 million individuals across the globe. The clinical manifestations persist chronically without a cure, and only a limited number of treatments demonstrate broad efficacy.
Tremors are identified by rhythmic oscillations of a body region, typically featuring a sinusoidal pattern [4–6]. Among all movement disorders, tremor reign as the most prevalent, afflicting over 20 million individuals globally, constituting around 3% of the general population. Tremor is a progressive symptom, resulting in heightened disability with advanced age. Similar to dystonias, tremors are persistent, subjecting patients to prolonged and stigmatizing impairment.
While dystonia and tremor are distinct disorders, they have close relationship. A particularly contentious relationship exists between neck dystonia and head tremor. Cervical dystonia (CD), the most prevalent form of dystonia, exhibits tremor-like characteristics due to intermittent, repetitive, and rapid movements of the neck. These tremor-like movements display irregularity and jerkiness, they are not really “tremor” but a jerky form of dystonia [7]. Dystonia may coexist with typical forms of head tremors, which typically manifest as sinusoidal patterns owing to comparable movement speeds in opposing directions [8–16].
Treatments of CD and related head tremor are limited. Though it’s well-established that botulinum neurotoxin (BoNT) significantly reduces muscle activity and head oscillations in up to 70% of cases, there are recognized limitations [17, 18]. BoNT injections are not effective in approximately 30% of patients. Higher doses of BoNT can markedly impact the quality of life by triggering difficulties in swallowing, speech, or breathing. Currently, the optimal selection of candidates for BoNT therapy hinges on trial and error, which can span months to years and is accompanied by high financial costs and the risk of severe side effects like dysphagia, dysarthria, and breathing problems. On the other hand, some types of CD are notoriously challenging to treat effectively. Some examples are predominant anterocollis with complex and varied movement patterns, and CD with prominent head tremor, a condition where oscillations are more prominent than abnormal postures [19–21] Our hypothesis centers on the idea that BoNT targets specific aspects of dystonia—abnormal turning versus irregular oscillations featuring jerky dystonia, or regular head tremor—with these aspects varying among patients. This inquiry revolves around which aspect of head oscillations BoNT modulates—whether amplitude, frequency, or irregularity. Does BoNT has different effects on various aspects of dystonia, and does this form the underlying reason why BoNT is highly effective in certain forms of dystonia, while it is ineffective in others, especially when head tremor is prominent. Elucidating the features of the complete dystonia phenotype and understanding how BoNT impacts these features will guide the selection of ideal candidates.
Methods
Participants
The study was approved by the Johns Hopkins University and Louis Stokes Cleveland VA Institutional Review Boards, and all participants gave informed consent before their involvement. Our goal was to examine effects of botulinum toxin injections on head tremor in CD. The head tremor is not always evident on clinical examination, often warranting objective measures [22–25]. Therefore we recruited 14 CD participants, whether or not they had head tremor during clinical examination. Head tremor was identified in 57% (n = 8 out of 14) of our cohort of CD patients when measured with search coils. These eight patients were further included the analysis reported in this current study. CD patients were excluded if they had known or presumed causes, broader involvement indicating segmental or generalized dystonia, and those who showed additional features suggestive of a more extensive neurodegenerative disorder. All participants had normal eye movements and visual acuity, corrected with lenses if required. Head movements were evaluated when their CD was at its peak, within a week before the scheduled botulinum toxin injection. We also evaluated head movements about 4 weeks after the BoNT injection, at its therapeutic peak. None of the patients were taking other medications for CD at the time of testing.
Experiment setup
The evaluation involved recording horizontal, torsional, and vertical head movements through a dual (three-axis) search coil (Skalar, Delft, Netherlands) fixed onto a bite bar (Figure 1). Participants were positioned within the magnetic coil frame so that the midpoint between the angles of the mouth coincided with the frame’s center (Figure 1). The trunk was stabilized by firm cushion mounted on a metal bar (Figure 1). Horizontal head movements were rotations around a vertical earth axis through the coil frame’s center (i.e., turning the head to the right or left, also referred to as torticollis). Vertical head movements constituted rotations around a horizontal axis parallel to the inter-aural line and passing through the coil frame’s center (i.e., head flexion and extension, also known as anterocollis or retrocollis). Torsional head movements occurred around a horizontal axis parallel to the naso-occipital axis of the head and through the coil frame’s center (i.e., tilting the head so that one ear moves towards a shoulder, also termed laterocollis). The search coil’s angular position with respect to magnetic fields was digitized at a rate of 1,000 Hz, and the data was processed to determine the head’s three-dimensional position [26]. The recordings were conducted in a dimly lit room, with participants wearing a headband containing a laser pointer (Figure 1). They were instructed to turn their heads toward a light-emitting diode (LED) targets placed at 0°, and either 10°, 20°, or 30° to the right or left. After repositioning their heads, they were asked to align the head-fixed laser with each LED target for about 40 s. MATLAB® software (The Mathworks™, Natick, MA) was used to analyze the three-dimensional head positions. Angular head velocity was derived from mathematical calculations of angular head position. Signal noise inherent to mathematical differentiation was removed using low-pass filtering and three-point averaging. Statistical analysis was carried out using the MATLAB® statistics toolbox.
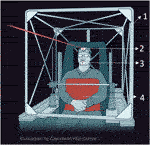
FIGURE 1. Schematic example of experiment setup (Images courtesy of The Cleveland FES Center). (1). search coil frame (2) head-fixed laser pointer (3) search coil affixed to the bitebar (4) body restraint system.
Analysis
Oscillatory head movements were analyzed after detrending the raw signal to remove the linear trend. Raw signal noise was eliminated through digital filtering, employing three-point averaging. Data from each axis underwent individual processing via a cycle-by-cycle analysis. A cycle was defined by initially eliminating bias from the detrended data (normalized amplitude = actual amplitude - mean amplitude). This rendered the cycle’s peaks positive and troughs negative. The intersection of the data trace with the abscissa (positive zero-crossing) marked the X-coordinate at which this happened, denoting the start and end of the cycle. Cycle frequency was derived from the inverse of the period of the cycle while the difference between the peak and trough indicated the peak-to-peak amplitude of the cycle.
Variance
We assessed the irregularity of tremor by quantifying variance. Variance of a time series signifies the dispersion of time series values around their average. Variance measurements from instances of regular and irregular head position time series are illustrated (Figures 2A–C). Irregular oscillatory head movements exhibited higher variance. As shown in Figure 2C, variance was greater in irregular head position compared to regular ones.
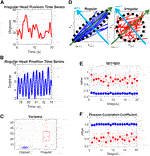
FIGURE 2. (A) Comparison of irregular movement, and (B) regular movement. (C) The boxplots of variance values show significantly larger values for irregular movement, red color, compared to regular movement, blue color. (D) Poincare maps depicting repetitive irregular, red color, and regular movements, blue color. A black ellipse is fitted and SD1, the standard deviations of the data points along the major semi-axis, green axis 1, and SD2, the standard deviations of the data points along the minor semi-axis, light blue axis 2, are calculated and compared. (E) The SD1/SD2 ratio represents the randomness in the time series. Lower values represent a more regular signal. (F) The Pearson Correlation Coefficient defines the linear correlation between a time series and its delayed version which means that regular time series data, has higher values compared to irregular time series data.
Poincaré analysis
Poincaré analysis was applied to the time series data by capturing its non-linear features [27]. The core principle involves identifying the likeness between an oscillatory cycle and the subsequent cycle that follows it, then the cycle following that, and so forth. The conjecture is that close by cycles will resemble each other more, and this feature gives rise to Poincaré parameters. Regular and rhythmic waveforms tend to display more resemblance in contrast to irregular and arrhythmic signals. To measure resemblance, the Poincaré algorithm produces maps of data points in the time series in relation to their subsequent data points (Figure 2D). These maps illustrate the evolution of the time series data concerning its variability. An ellipse is fitted to the plot, and standard deviations along the minor and major semi-axes of the fitted ellipse are calculated. These parameters define short-term and long-term variability in the time series, and their ratio signifies the randomness within the time series. Examples of Poincaré plots, illustrating repetitive movements in regular and irregular head position time series, are shown in Figure 2D. In each case, a black ellipse is fitted, and SD1 represents the standard deviation of data points along the major semi-axis (green axis 1), indicating short-term variability. SD2 is the standard deviation of data points along the minor semi-axis (light blue axis 2), reflecting long-term variability. In a regular time series, most data points fall within the fitted ellipse, leading to a relatively small SD1 value and a relatively large SD2 value. The SD1/SD2 ratio denotes the randomness in the time series. A more regular time series is associated with a smaller ratio value. Poincaré ratio values that evolve over the cycles’ proximity are depicted in Figure 2E, showing larger values for irregular time series (blue) and smaller values for regular time series (red). Another parameter examined in Poincaré analysis is the Pearson Correlation Coefficient, ρ. This value signifies the linear correlation between a time series and its delayed version, indicating that regular time series data exhibit higher ρ values compared to irregular time series data. Examples of ρ are shown in Figure 2F.
Results
This study aimed to investigate how BoNT affects oscillatory head movements in individuals with CD. Fourteen patients with CD were screened to find head tremor with dystonia; eight patients (57%) exhibited observable oscillatory head movements when assessed with instrumented measures using search coils. These eight patients were subsequently included in the experiment. The study’s focus centered around addressing two specific questions:
1) To examine the effects of BoNT on amplitude, frequency, and regularity of oscillatory head movements in CD.
2) To examine the effects of BoNT on head-on-trunk position dependence of oscillatory head movements.
Question 1. To examine the effects of BoNT on amplitude, frequency, and regularity of oscillatory head movements.
In the upper panel of Figure 3, the measures of head positions in three exemplary patients are displayed as they look at targets positioned on their left (P7), right (P6), and center (P8). The measurements are presented in degrees, where positive values indicate rightward direction, negative values indicate leftward direction, and zero signifies the central direction. Within this panel, the head position measurements before and after BoNT injection are shown for each patient with blue and red lines, respectively.
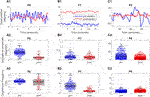
FIGURE 3. (A1,B1,C1) Raw data depicting the head position over time for three CD patients. The x-axis shows the time in seconds and the y-axis shows the head position in degrees. (A2,B2,C2) The effect of Botox injection on amplitude and (A3,B3,C3) frequency of oscillations for the patients. The blue color represents the head position measurements taken before Botox injection and red color represents the head position measurements taken after the Botox injection. The vertical axis shows the boxplots of the amplitude and frequency values. The horizontal black line in the middle of each box is the median of the data and the whiskers indicate the 25th and 75th percentiles. The horizontal axis provides the comparison between pre-injection (blue color) and post-injection (red color) values.
Three distinct trends in regularity change can be observed among these patients: prior to BoNT injection, patient P6’s head oscillations in Figure 3A1 exhibited regular sinusoidal patterns with comparable frequencies and varying amplitudes. However, after the injection, these oscillations became irregular, featuring fluctuating frequencies and reduced amplitudes. Conversely, the oscillations of patient P7 in Figure 3B1 displayed minimal alterations in terms of regularity, with their amplitudes decreasing to some extent while higher frequencies were attenuated. Finally, patient P8’s irregular oscillations in Figure 3C1 prior to BoNT injection became more regular with diminished amplitudes and consistent frequencies after the injection.
We assessed the amplitude and frequency of individual oscillations to look more closely into the effects of BoNT on the amplitude and frequency of head movements. The amplitude and frequency box plots for these single oscillations are provided in the middle and lower panels of Figure 3, respectively. Similarly, blue color signifies measurements taken before BoNT injection, while the red color represents the measurements taken after injection.
Upon close examination, three different post-injection effects were evident. The Patient P6 (Figure 3A2) had reduction in the amplitude of sinusoidal head oscillations, but there was no change in frequency (Figure 3A3; Supplementary Table S1). Prior to BoNT injection, P6’s had regular and sinusoidal oscillations, which became less regular with varying amplitude and inconsistent frequency after injections (Figure 3A2). An example of patient P7 (Figure 3B1) had elimination of higher frequency oscillations following BoNT injection (Figure 3B3), while amplitudes remained unaffected (Figure 3B2; Supplementary Table S1). The irregular nature of oscillations persisted (Figure 3B1). Similarly, BoNT diminished head tremor amplitude in patient P8 (Figures 3C1, C2) but there was an increase in the frequency (Figure 3C3; Supplementary Table S1). There was improved regularity and less frequency variability in P8’s head oscillations after BoNT injections.
In summary, three trends emerge concerning the regularity of head tremor post BoNT injection: 1) a transition from irregular to regular, exemplified by P8 in Figure 3C; 2) a shift from regular to irregular, as observed in the case of P6 in Figure 3A; and 3) an absence of change, similar to what’s seen in P7 in Figure 3B. To quantify these shifts, we used Poincaré analysis on the head position measurements and juxtapose Poincaré parameters before and after the BoNT injection. This involves segmenting the time series of head position measurements into distinct oscillations, followed by Poincaré analysis of these individual oscillations. Figure 4 displays the Pearson’s correlation coefficient values for all patients based on the Poincaré steps. The horizontal axis signifies Poincaré steps denoted as “k,” while the vertical axis depicts Pearson’s correlation coefficient values before and after BoNT injection in blue and red colors, respectively. Each “k” represents the interval between single oscillations. For instance, at “k = 5,” the first oscillation “x1” is contrasted with the 6th oscillation “x6,” and the corresponding correlation coefficient value is computed. The filled circles denote mean values, and the whiskers represent error bars indicating a 95% confidence interval at each step.
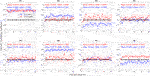
FIGURE 4. Graph of Pearson correlation coefficient of Poincare plot parameter for CD patients before and after the Botox injection. Blue circles represent pre-injection, and red circles represent post-injection. The vertical axis shows the Pearson correlation coefficient value, and the horizontal axis shows the steps. The vertical lines on the graphs represent 95% confidence interval at each step.
The correlation coefficient serves as a marker for regularity within the time series, with higher values signifying a greater level of regularity. Several patterns appeared: 1) heightened regularity after botulinum toxin injection for P3, P4, P5, and P8; 2) diminished regularity post-injection for P2 and P6; and 3) sustained regularity for P1 and P7. Furthermore, it is evident that post-injection correlation coefficient values cluster around 0.4 for all patients, excluding P1, whereas these values showed a range of levels before BoNT injection. After excluding the outlier (P1), mean and standard deviation of offset values before injection was 0.41 ± 0.19. After injection the mean value of offset remained about the same, but the standard deviation significantly reduced (0.45 ± 0.04).
The SD1/SD2 ratio reflects the randomness of the head position measurements, with lower ratios indicating more regular head tremors. Figure 5 compares the ratios for all patients before and after injection. Like the correlation coefficient, post-injection values cluster around 0.6, whereas they were more dispersed prior to BoNT injection. Randomness in head tremor oscillations is diminished for P3, P4, P5, and P8, augmented for P2 and P6, and did not change for P1 and P7. This corroborates the findings derived from the correlation coefficient in Figure 4. As noted in Figure 4, for correlation coefficients, pre-injection values of offset had a higher spread (before: 0.83 ± 0.35); but the spread of the offset was significantly reduced after BoNT injection (after: 0.6830 ± 0.036).
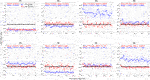
FIGURE 5. Graph of SD1/SD2 ratio of Poincare plot parameter for CD patients before and after the Botox injection. Blue circles represent pre-injection, and red circles represent post-injection. The vertical axis shows the Pearson correlation coefficient value, and the horizontal axis shows the steps. The vertical lines on the graphs represent 95% confidence interval at each step.
Regarding the first question—the effect of BoNT injection on the regularity of the head oscillations -- the Poincaré analysis indicates that BoNT injection did impact their regularity in some patients. The toxin imbues a certain regularity referred to as a “set point,” which remains consistent across all patients. If pre-injection head oscillations are more irregular than this “set point,” they tend to become more regular. Conversely, if pre-injection oscillations are more regular than the “set point,” they shift towards increased irregularity.
The Poincaré analysis leads to an important insight into the nature of head oscillations in CD. It shows that these oscillations exhibit varying, random shapes, and the degree of randomness does not change although the time gap between two compared oscillations increases. In other words, the difference in shapes between the first (x1) and sixth (x6) oscillations is no different from the difference between the first (x1) and twentieth (x20) oscillation cycles. This observation was quantitatively assessed by analyzing the slope of a linear trend within the scatter plots in Figures 4, 5. This scatter plot compared the Pearson correlation coefficient and Poincaré steps, as well as the SD1/SD2 ratio and Poincaré steps. The slope of this trend would be greater if the disparity in shape between cycles increased with a greater time gap between them, but it would be zero if the randomness of the shapes remained constant. Specifically, before the administration of BoNT injections, the slopes comparing Pearson correlation coefficients and Poincaré steps were measured at 0.002 ± 0.0006, and after BoNT injections, they remained quite similar at 0.002 ± 0.0009. Likewise, the slopes comparing SD1/SD2 ratio and Poincaré steps were 0.002 ± 0.0009 before BoNT injection and about the same (0.003 ± 0.002) after BoNT injection. Furthermore, the variations between patients underscore an important observation—the effects of injection might hinge on the injection site and possibly the baseline disease phenomenology. The subsequent section explores the influence of these changes based on the head-on-trunk orientation.
To summarize, we find three distinct trends in the changes in the regularity of the head oscillations induced by BoNT among CD patients—head oscillations become irregular post-injection, oscillations remained relatively consistent in regularity, and the irregular oscillations transform into a more regular pattern. Poincaré analysis quantified these shifts in regularity, highlighting that BoNT brings regularity to a “set point.” Moreover, the analysis reveals that head oscillations exhibit random shapes that remain consistent regardless of the time interval between cycles.
Question 2. To examine the effects of botulinum toxin on head-on-trunk position dependence of oscillatory head movements:
The primary characteristic of CD is its dependence on the head’s position relative to the trunk, particularly evident in head oscillations. Usually, the head oscillations are minimal in one specific head orientation, known as the null position, but increase in intensity as the head deviates further from this null orientation. In this section, we investigate how BoNT injections affect the relationship between head-on-trunk position and head oscillations. We begin by examining the amplitude and frequency of individual oscillations across various head orientations, as illustrated in Figures 6A, B, respectively. These figures display the mean values along with a 95% confidence interval for both amplitude and frequency at each head orientation. Figure 6A reveals a notable decrease in the amplitude of oscillations following BoNT injections for patients P1, P5, P6, and P8, across all measured head orientations. This reduction is most pronounced for head orientations previously associated with high-intensity pre-injection oscillations. This effect is most striking in the cases of P3, P4, and P7, where changes in head oscillations were only observed in head orientations that initially had high intensity oscillations pre-injection. Conversely, the effects were minimal in the cases of P2 and P7, where the intensity of head oscillations pre-injection was modest. Regarding the frequency of oscillations (Figure 6B), there was a decrease across all head orientations for all patients except for P1 and P5.
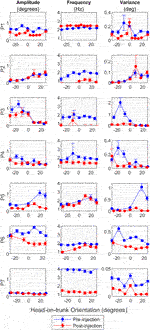
FIGURE 6. (A) The effect of BoNT injection on the amplitude of oscillations for all CD patients where multiple head on trunk orientations were measured. The vertical axis shows the oscillation amplitude value in degrees, and the horizontal axis shows the head orientation in degrees. Blue circles represent mean values for pre-injection, and red circles represent mean values for post-injection. The vertical lines on the graphs are 95% confidence interval at each head orientation. (B) The effect of BoNT injection on the frequency of oscillations for CD patients where head on trunk orientation dependence was measured. The vertical axis shows the oscillation frequency value in Hz, and the horizontal axis shows the head orientation in degrees. Blue circles represent mean values for pre-injection, and red circles represent mean values for post-injection. The vertical lines on the graphs are 95% confidence interval at each head orientation. (C) The effect of BoNT injection on the variance of oscillations amplitude for CD patients where head on trunk orientation dependence was measured. The vertical axis shows the variance, and the horizontal axis shows the head orientation in degrees. Blue circles represent mean values for pre-injection, and red circles represent mean values for post-injection. The vertical lines on the graphs are 95% confidence interval at each head orientation.
To further investigate the relationship between head-on-trunk position and BoNT injection effects, we analyzed the variance in head position amplitude, as shown in Figure 6C. There is a reduction in variance except in the case of P2, who consistently showed low-intensity head oscillations at baseline. In summary, the impact of BoNT on head oscillations is most pronounced in head orientations in which high intensity head oscillations were triggered before treatment.
To summarize, the results show a considerable reduction in head oscillation amplitude after BoNT injections, especially in head orientations associated with intense pre-injection oscillations. The frequency of oscillation decreased in all patients except two. The variance in head position amplitude decreased overall, except for one patient who had low-intensity head oscillations at baseline.
Discussion
The results of this study provide valuable insights into the effects of BoNT on oscillatory head movements in individuals with CD. BoNT injections led to three types of changes: a transition from irregular to more regular oscillations, a shift from regular to more irregular oscillations, or unchanged regularity. The Poincaré analysis further supported these findings by quantifying regularity using correlation coefficient values. It indicated that BoNT injection affects head oscillations by altering their regularity. A “set point” of regularity is established by BoNT that tends to regularize irregular tremors, but it may also make regular tremors more irregular. The intriguing finding in this study is that across all patients, prior to BoNT treatment, head oscillations displayed a variable irregularity. In other words, one cycle of the oscillation could not predict the shape of subsequent cycles, suggesting that the oscillations were random in shape. Furthermore, the BoNT did not alter this trend of randomness. These results highlight that neck oscillations studied here do not satisfy the definition of tremor (although traditionally called dystonic “tremor”) [3, 5–7]. The tremor by definition is regular, rhythmic, and back-and-forth oscillatory cycles that allow for predicting the shape of subsequent cycles based on the shape of one cycle [5, 6].
Another important observation was that BoNT affected the overall regularity of the oscillations. However, the effect of BoNT on the oscillations was variable among patients and it was independent of the baseline irregularity. In essence, if the oscillatory cycle was highly irregular before treatment, its irregularity decreased after BoNT administration, whereas if it was highly regular, its regularity decreased after BoNT injection. These results were measured using Poincaré analysis, which involved comparing the correlation Poincaré estimations of the oscillation shapes before and after BoNT treatment.
We speculate two co-existing phenomenology to explain these findings. One, there is a central oscillator that may be inherently similar in all CD patients but receives varied input from other feedback sources. These feedback sources can alter the oscillatory characteristics and introduce irregularity. The nature of the feedback influence could be determined by the type of connectivity pattern with the oscillator; some making it regular some making it irregular. In other words, CD in some patients involves neck muscles that influence oscillator to make it more regular while in other instances the oscillator may become irregular. Treatment of CD with BoNT may revert the oscillator at the “set point,” returning the oscillations to an identical regularity. In addition, the neck oscillations in CD may be under cerebellar, basal ganglia, and neck proprioceptive influence. BoNT may directly affects proprioceptive modulation but it may not directly affect the cerebellum. Nevertheless, the cerebellum may still contribute to the oscillatory instability independent of the instability caused by proprioceptive feedback.
Our results are supports the idea of two separate pathophysiology contributing to head oscillations in CD, one originating from the cerebellum and basal ganglia and the other from proprioception. This concept is consistent with the notion that dystonia is a network disorder [28–30]. The results also support the idea that although dystonia and tremor often coexist, they may still represent distinct entities with different underlying pathophysiological mechanisms [31]. Recent physiological studies measuring single-neuron activity in CD participants have further emphasized these distinctions. For instance, individuals with irregular head oscillations combined with dystonia displayed distinct pallidal physiology compared to those with pure dystonia or jerky head oscillations [31].
Contemporary literature over the last one decade has increasingly supported the role of mesencephalic neural integrator for the control of the head position in CD [23, 25, 32–36]. According to the network model in CD, the impairment anywhere in the network, even outside of the integrator, may lead to deficits in the feedback dependent neural integration. There is increasing evidence for the involvement of cerebellum, proprioception, and basal ganglia, as three separate sources of the feedback in the network model for CD [28–32, 37–39]. The cerebellar role in dystonia is supported when the oscillations coexisting with dystonia have sinusoidal features as seen in tremor that is also thought to be related to cerebellar deficits. Our results support the role of proprioception as an independent source of feedback to the integrator, and effect of BoNT on the integrator function.
These findings also support the notion that head oscillations and CD may not effectively respond to a single treatment modality, and a combination of approaches may be necessary. Some types of head oscillations, particularly those more influenced by proprioception, could benefit from BoNT treatment, while others may require pharmacotherapy for tremor or even deep brain stimulation. The combination of deep brain stimulation and BoNT for the treatment of tremor and dystonia is a practice that aligns with these results and previous physiological experiments.
In conclusion, this study provides insights into the complex relationship between BoNT and head tremors in CD patients. It highlights the need for personalized treatment approaches, considering individual variations in tremor characteristics and head orientations. Understanding how BoNT influences the regularity and amplitude of head oscillations is a crucial step in optimizing its therapeutic benefits for CD patients.
Data availability statement
Deidentified data will be available to interested parties after completing all necessary documentation with Johns Hopkins University and U.S. Department of Veterans’ Affairs. Requests to access the datasets should be directed to aasefshaikh@gmail.com.
Ethics statement
The studies involving humans were approved by Johns Hopkins University and Louis Stokes Cleveland VA Institutional Review Board. The studies were conducted in accordance with the local legislation and institutional requirements. The participants provided their written informed consent to participate in this study.
Author contributions
HA: analyzed data, conceptualized analysis and interpretation, wrote and edited the paper. HJ and DZ: conceptualized analysis and interpretation, edited the paper. AS: collected data, analyzed data, conceptualized analysis and interpretation, wrote and edited the paper. All authors contributed to the article and approved the submitted version.
Funding
AS was supported by the Career Development Grant from the American Academy of Neurology, George C. Cotzias Memorial Fellowship, Network Models in Dystonia grant from the Dystonia Medical Research Foundation, Department of VA Merit Review (I01CX002086, I01RX003676), Department of VA SPiRE (I21RX003878) CareSource Ohio Community Partnership Grant, and philanthropic funds to the Department of Neurology at University Hospitals (Penni and Stephen Weinberg Chair in Brain Health and Allan Woll Fund).
Conflict of interest
AS serves on speaker bureau for Accorda Pharmaceuticals, and Abbott Neuroscience.
The remaining authors declare that the research was conducted in the absence of any commercial or financial relationships that could be construed as a potential conflict of interest.
Supplementary material
The Supplementary Material for this article can be found online at: https://www.frontierspartnerships.org/articles/10.3389/dyst.2024.12347/full#supplementary-material.
References
1. Evatt, ML, Freeman, A, and Factor, S. Adult-onset dystonia. Handb Clin Neurol (2011) 100:481–511. doi:10.1016/B978-0-444-52014-2.00037-9
2. Jinnah, HA, Berardelli, A, Comella, C, Defazio, G, DeLong, M, Factor, S, et al. The focal dystonias: current views and challenges for future research. Mov Disord (2013) 7:926–43. doi:10.1002/mds.25567
3. Albanese, A, Bhatia, K, Bressman, SB, Delong, MR, Fahn, S, Fung, VS, et al. Phenomenology and classification of dystonia: a consensus update. Mov Disord (2013) 28(7):863–73. doi:10.1002/mds.25475
5. Deuschl, G, Bain, P, and Brin, M. Consensus statement of the movement disorder society on tremor. ad hoc scientific committee. Mov Disord (1998) 13(Suppl. 3):2–23. doi:10.1002/mds.870131303
6. Bhatia, KP, Bain, P, Bajaj, N, Elble, RJ, Hallett, M, Louis, ED, et al. Consensus statement on the classification of tremors. From the task force on tremor of the international Parkinson and movement disorder society. Mov Disord (2018) 33(1):75–87. doi:10.1002/mds.27121
7. Fahn, S. The varied clinical expressions of dystonia. Neurol Clin (1984) 2:541–54. doi:10.1016/s0733-8619(18)31090-9
8. Chan, J, Brin, MF, and Fahn, S. Idiopathic cervical dystonia: clinical characteristics. Mov Disord (1991) 6:119–26. doi:10.1002/mds.870060206
9. Jankovic, J, Leader, S, Warner, D, and Schwartz, K. Cervical dystonia: clinical findings and associated movement disorders. Neurology (1991) 41:1088–91. doi:10.1212/wnl.41.7.1088
10. Deuschl, G, Heinen, F, Guschlbauer, B, Schneider, S, Glocker, FX, and Lucking, CH. Hand tremor in patients with spasmodic torticollis. Mov Disord (1997) 12:547–52. doi:10.1002/mds.870120411
11. Pal, PK, Samii, A, Schulzer, M, Mak, E, and Tsui, JK. Head tremor in cervical dystonia. Can J Neurol Sci (2000) 27:137–42. doi:10.1017/s0317167100052240
12. Schweinfurth, JM, Billante, M, and Courey, MS. Risk factors and demographics in patients with spasmodic dysphonia. Laryngoscope (2002) 112:220–3. doi:10.1097/00005537-200202000-00004
13. Shatunov, A, Sambuughin, N, Jankovic, J, Elble, R, Lee, HS, Singleton, AB, et al. Genomewide scans in North American families reveal genetic linkage of essential tremor to a region on chromosome 6p23. Brain (2006) 129:2318–31. doi:10.1093/brain/awl120
14. Godeiro-Junior, C, Felicio, AC, Aguiar, PC, Borges, V, Silva, SM, and Ferraz, HB. Head tremor in patients with cervical dystonia: different outcome? Arq Neuropsiquiatr (2008) 66:805–8. doi:10.1590/s0004-282x2008000600005
15. Hedera, P, Phibbs, FT, Fang, JY, Cooper, MK, Charles, PD, and Davis, TL. Clustering of dystonia in some pedigrees with autosomal dominant essential tremor suggests the existence of a distinct subtype of essential tremor. BMC Neurol (2010) 10:66. doi:10.1186/1471-2377-10-66
16. Louis, ED, Hernandez, N, Alcalay, RN, Tirri, DJ, Ottman, R, and Clark, LN. Prevalence and features of unreported dystonia in a family study of "pure" essential tremor. Parkinsonism Relat Disord (2013) 19:359–62. doi:10.1016/j.parkreldis.2012.09.015
17. Sethi, KD, Rodriguez, R, and Olayinka, B. Satisfaction with botulinum toxin treatment: a cross-sectional survey of patients with cervical dystonia. J Med Econ (2012) 15(3):419–23. doi:10.3111/13696998.2011.653726
18. Zoons, E, Dijkgraaf, MG, Dijk, JM, van Schaik, IN, and Tijssen, MA. Botulinum toxin as treatment for focal dystonia: a systematic review of the pharmaco-therapeutic and pharmaco-economic value. J Neurol (2012) 259:2519–26. doi:10.1007/s00415-012-6510-x
19. Jinnah, HA. The dystonias. Continuum (Minneap Minn) (2019) 25(4):976–1000. doi:10.1212/CON.0000000000000747
20. Jinnah, HA, Comella, CL, Perlmutter, J, Lungu, C, and Hallett, MDystonia Coalition Investigators. Longitudinal studies of botulinum toxin in cervical dystonia: why do patients discontinue therapy? Toxicon (2018) 147:89–95. doi:10.1016/j.toxicon.2017.09.004
21. Merola, A, Dwivedi, AK, Shaikh, AG, Tareen, TK, Da Prat, GA, Kauffman, MA, et al. Head tremor at disease onset: an ataxic phenotype of cervical dystonia. J Neurol (2019) 266(8):1844–51. doi:10.1007/s00415-019-09341-w
22. Beylergil, SB, Singh, AP, Zee, DS, Jinnah, HA, and Shaikh, AG. Relationship between jerky and sinusoidal oscillations in cervical dystonia. Parkinsonism Relat Disord (2019) 66:130–7. doi:10.1016/j.parkreldis.2019.07.024
23. Shaikh, AG, Wong, A, Zee, DS, and Jinnah, HA. Why are voluntary head movements in cervical dystonia slow?. Parkinsonism Relat Disord (2015) 21(6):561–6. doi:10.1016/j.parkreldis.2015.03.005
24. Shaikh, AG, Wong, AL, Zee, DS, and Jinnah, HA. Keeping your head on target. J Neurosci (2013) 33(27):11281–95. doi:10.1523/JNEUROSCI.3415-12.2013
25. Shaikh, AG, Zee, DS, and Jinnah, HA. Oscillatory head movements in cervical dystonia: dystonia, tremor, or both?. Mov Disord (2015) 30(6):834–42. doi:10.1002/mds.26231
26. Bergamin, O, Zee, DS, Roberts, DC, Landau, K, Lasker, AG, and Straumann, D. Three-dimensional Hess screen test with binocular dual search coils in a three-field magnetic system. Invest Ophthalmol Vis Sci (2001) 42(3):660–7.
27. Woo, MA, Stevenson, WG, Moser, DK, Trelease, RB, and Harper, RM. Patterns of beat-to-beat heart rate variability in advanced heart failure. Am Heart J (1992) 123(3):704–10. doi:10.1016/0002-8703(92)90510-3
28. Prudente, CN, Hess, EJ, and Jinnah, HA. Dystonia as a network disorder: what is the role of the cerebellum?. Neuroscience (2014) 260:23–35. doi:10.1016/j.neuroscience.2013.11.062
29. Berman, BD, and Jinnah, HA. Dystonia: five new things. Neurol Clin Pract (2015) 5:232–40. doi:10.1212/CPJ.0000000000000128
30. Neychev, VK, Gross, R, Lehericy, S, Hess, EJ, and Jinnah, HA. The functional neuroanatomy of dystonia. Neurobiol Dis (2011) 42:185–201. doi:10.1016/j.nbd.2011.01.026
31. Sedov, A, Usova, S, Semenova, U, Gamaleya, A, Tomskiy, A, Beylergil, SB, et al. Pallidal activity in cervical dystonia with and without head tremor. Cerebellum (2020) 19(3):409–18. doi:10.1007/s12311-020-01119-5
32. Sedov, A, Semenova, U, Usova, S, Tomskiy, A, Crawford, JD, Jinnah, HA, et al. Implications of asymmetric neural activity patterns in the basal ganglia outflow in the integrative neural network model for cervical dystonia. Prog Brain Res (2019) 249:261–8. doi:10.1016/bs.pbr.2019.03.030
33. Sedov, A, Usova, S, Semenova, U, Gamaleya, A, Tomskiy, A, Crawford, JD, et al. The role of pallidum in the neural integrator model of cervical dystonia. Neurobiol Dis (2019) 125:45–54. doi:10.1016/j.nbd.2019.01.011
34. Sedov, A, Popov, V, Shabalov, V, Raeva, S, Jinnah, HA, and Shaikh, AG. Physiology of midbrain head movement neurons in cervical dystonia. Mov Disord (2017) 32(6):904–12. doi:10.1002/mds.26948
35. Shaikh, AG, Zee, DS, Crawford, JD, and Jinnah, HA. Cervical dystonia: a neural integrator disorder. Brain (2016) 139:2590–9. doi:10.1093/brain/aww141
36. Shaikh, AG, Wong, AL, Zee, DS, and Jinnah, HA. Keeping your head on target. J Neurosci (2013) 33:11281–95. doi:10.1523/JNEUROSCI.3415-12.2013
37. Pyatka, N, Sedov, A, Walter, BL, Jinnah, HA, and Shaikh, AG. Tremor in chronic inflammatory demyelinating polyneuropathy: proof of unifying network model for dystonia. Prog Brain Res (2019) 249:285–94. doi:10.1016/bs.pbr.2019.03.032
38. Neychev, V, Fan, X, Mitev, VI, Hess, EJ, and Jinnah, HA. The basal ganglia and cerebellum interact in the expression of dystonic movement. Brain (2008) 131:2499–509. doi:10.1093/brain/awn168
Keywords: dystonia, tremor, torticollis, botulinum neurotoxin, dystonic tremor
Citation: Agharazi H, Jinnah HA, Zee DS and Shaikh AG (2024) Effects of botulinum neurotoxin on regularity of head oscillations in cervical dystonia. Dystonia 3:12347. doi: 10.3389/dyst.2024.12347
Received: 31 October 2023; Accepted: 16 February 2024;
Published: 06 March 2024.
Edited by:
Aparna Wagle Shukla, University of Florida, United StatesCopyright © 2024 Agharazi, Jinnah, Zee and Shaikh. This is an open-access article distributed under the terms of the Creative Commons Attribution License (CC BY). The use, distribution or reproduction in other forums is permitted, provided the original author(s) and the copyright owner(s) are credited and that the original publication in this journal is cited, in accordance with accepted academic practice. No use, distribution or reproduction is permitted which does not comply with these terms.
*Correspondence: Aasef G. Shaikh, aasef.shaikh@case.edu