- 1Institute of Molecular Biomedicine, Faculty of Medicine, Comenius University, Bratislava, Slovakia
- 2Institute of Virology, Biomedical Research Center, Slovak Academy of Sciences, Bratislava, Slovakia
- 3Institute of Pathophysiology, Faculty of Medicine, Comenius University, Bratislava, Slovakia
The severe acute respiratory syndrome coronavirus 2 (SARS-CoV-2) infection has a highly variable course that is dependent on the host immune system reaction. Lung tissue damage, endothelial dysfunction, and microthrombosis in severe COVID-19 is linked to neutrophilia and the production of neutrophil extracellular traps (NETs). Previous studies have shown that NETs are involved in the pathology of COVID-19 and that the virus itself induces NET formation, although the underlying mechanisms are not clear. In this study, we aimed to investigate the induction of NETs by SARS-CoV-2 in vitro. We have found that both, infectious and heat-inactivated virus induce NETs formation. Surprisingly, cell culture media derived from uninfected Vero cells exhibit similar potency. This suggests that NET inducers other than the virus might be involved. Mitochondria released from dying cells during SARS-CoV-2 infection acting as damage-associated molecular patterns (DAMPs) were identified as potential contributors to neutrophil activation and NET formation. Our findings point to an important source of bias when analyzing NETs induction by SARS-CoV-2 in vitro, but also the immune reaction to viruses in general. Further implications for the understanding of COVID-19 pathogenesis remain to be elucidated.
Introduction
In the early stages of the COVID-19 pandemic, it was observed that severe SARS-CoV-2 infection results in the destruction of lung tissue and impaired oxygenation. Initial treatment approaches utilizing mechanical ventilation were, however, met with high mortality, highlighting the need for a better understanding of COVID-19 pathology (Auld et al., 2020). Subsequent studies associated thrombosis with disease severity and identified neutrophilia as a marker of poor outcome (Klok et al., 2020; Lagunas-Rangel, 2020). This led to the hypothesis that the neutrophil extracellular traps (NETs) cause thrombi formation, thereby reducing the efficiency of artificial ventilation. NETs are web-like structures composed of DNA and antimicrobial proteins, that are induced in response to both pathogenic (bacteria, fungi, and viruses) as well as sterile (crystals, immune complexes, mitochondria, and platelets) stimuli (Brinkmann et al., 2004; Papayannopoulos, 2018). While the primary function of NETs is host defense, their excessive production is implicated in disseminated intravascular coagulation during systemic inflammation. Multiple studies have also observed an association between increased NET production and the severity of COVID-19 (Zuo et al., 2020). In order to improve the treatment not just for COVID-19 patients, but also for other viral respiratory tract infections, a comprehensive understanding of the mechanisms underlying NET induction is crucial. Studies have already shown that both infectious and heat-inactivated SARS-CoV-2 induce the formation of NETs in isolated neutrophils; however, the in vitro conditions often do not reflect the complex situation that is present in vivo (Arcanjo et al., 2020; Veras et al., 2020). In this work, we analyze the potency of both infectious and heat-inactivated cell cultivation media containing SARS-CoV-2 to induce NET formation, with a focus on characterizing the surrounding milieu that modulates neutrophil response.
Methods
Neutrophil isolation
Healthy adult donors volunteered and consented to blood collection under ethical approval given by the Ethics Committee of the University Hospital Bratislava (workplace Ruzinov under number EK 218/2020). Blood was collected by venous puncture into heparinized tubes and neutrophils were isolated using 1-Step Polymorphs (Accurate Chemical & Scientific Corp, United States) and resuspended in RPMI 1640 with 10% FBS (PAN-Biotech, Germany).
SARS-CoV-2 preparation
The SARS-CoV-2 cell culture isolate Slovakia/SK-BMC5/20201 was used in the experiments. The strain represents the virus that was circulating in Europe in spring 2020 and carries the Spike D614G mutation (lineage B.1). The virus was cultured on the Vero E6 cell line (ECACC Cat# 85020206, RRID:CVCL_0574) in complete DMEM medium with 5% FBS (Gibco, United Kingdom). The virus titer was determined to be 2 × 106 plaque forming units (PFU)/ml using a plaque assay described previously (Kovacech et al., 2022). The initial experiment, where the formation of NETs was analyzed by fluorescence microscopy (Figure 1A), was performed with media collected from uninfected and virus-infected Vero E6 cells under biosafety level 3 conditions. The following experiments, such as quantification of extracellular mitochondria or analysis of NET formation using live-cell microscopy (Figures 1B–E, 2, respectively), were performed with media collected from uninfected and virus-infected Vero cells that were heat-inactivated (60°C for 30 min). Heat inactivation was verified using a plaque formation assay and was found to be completely efficient.
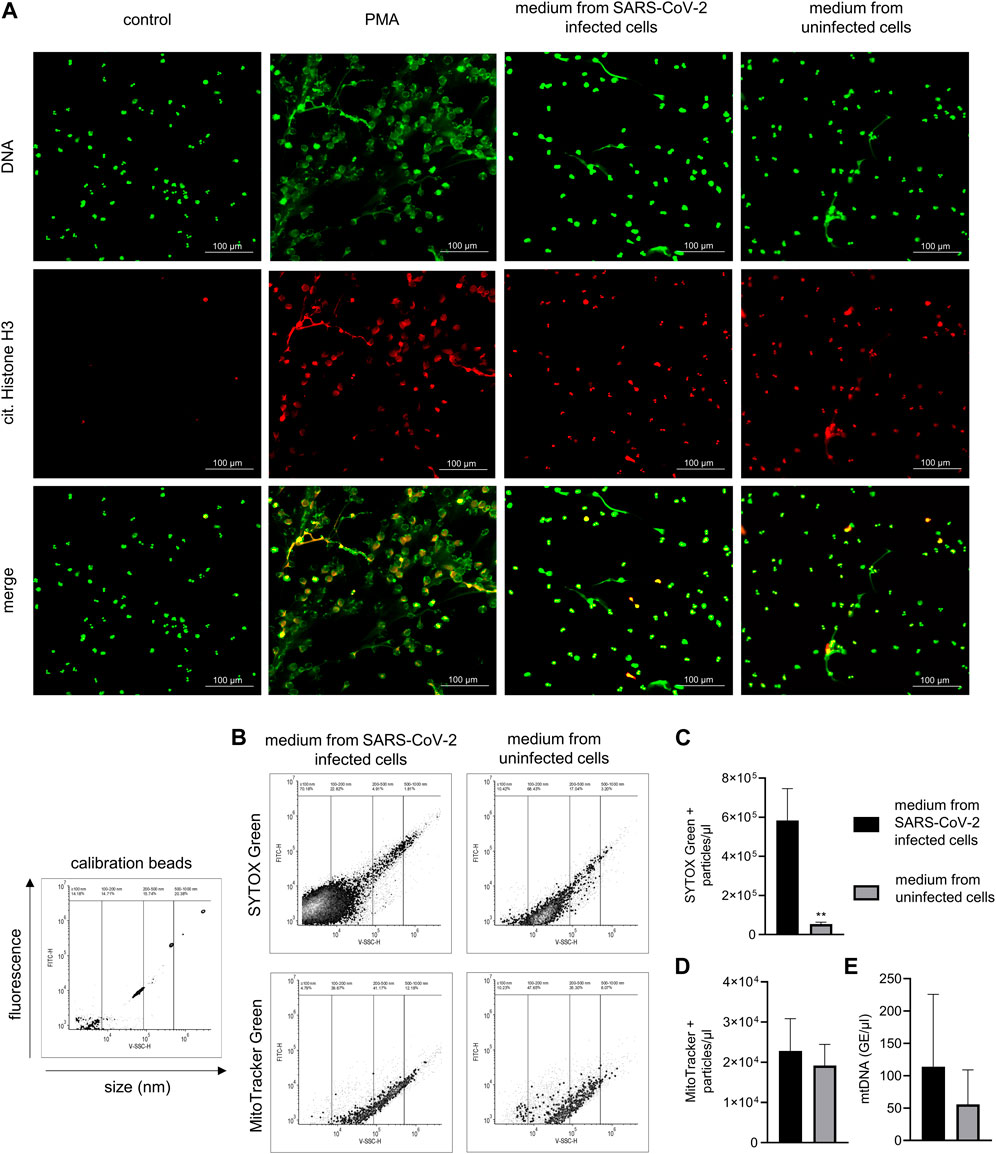
Figure 1. NETs production in response to SARS-CoV-2–infected and uninfected Vero E6 cell media and analysis of media content. (A) Representative images of NETs formation in response to media collected from SARS-CoV-2–infected Vero E6 cells containing live virus (MOI 1), uninfected Vero E6 cells, and 100 nM PMA incubated with neutrophils for 3 h. A pure medium was used as a control. NETs are identified according to positive staining for citrullinated histone H3 (red) and DNA (green). (B) Calibration of flow cytometry for the detection of submicron particles with representative images of nucleic acid–associated particles detected by SYTOX Green and MitoTracker Green. (C,D) Quantity of nucleic acid–and mitochondria-associated particles in heat-inactivated SARS-CoV-2–infected and uninfected Vero E6 cell media, analyzed using flow cytometry. (E) Analysis of mtDNA quantity by qPCR, expressed as concentration of genomic equivalents/μL. All analyses were independently repeated three times. ** = p < 0.01. Two-tailed T-test.
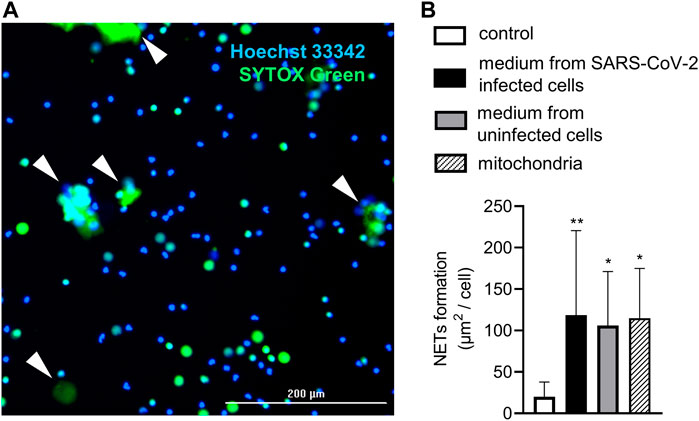
Figure 2. Live-cell imaging of NET formation. (A) Representative image from live-cell imaging. NETs marked with white arrows are identified according to positivity for SYTOX Green and size >20 µm. (B) Relative NET formation in response to mitochondria (20 mitochondria particles/cell) and heat-inactivated media from SARS-CoV-2–infected (MOI 10) and uninfected Vero E6 cells. A pure cultivation medium was used as a control. n = 11, ** = p < 0.01, * = p < 0.05. One-way ANOVA followed by Dunnet’s multiple comparison test.
Mitochondria isolation and quantification
Placental tissue was homogenized in STE buffer (250 mM saccharose, 2 mM EGTA, 5 mM Tris-HCl, pH = 7.4) using a Dounce homogenizer, and mitochondria were isolated by performing a series of three differential centrifugations at 500 × g for 3 min at 4°C and 8,000 × g for 10 min at 4°C. The purity and quantity of mitochondria were assessed by qPCR using primers designed to amplify part of the D-loop hypervariable region of mtDNA (forward: 5′-CATAAAAACCCAATCCACATCA-3′, reverse: 5′-GAGGGGTGGCTTTGGAGT-3′) and part of the human globin gene to quantify ncDNA (forward: 5′-GCTTCTGACACAACTGTGTTCACTAGC-3′, reverse: 5′-CACCAACTTCATCCACGTTCACC-3′) on a QTower3 (Analytik Jena GmbH, Germany) using the Advanced Universal SYBR Green Supermix (Bio-Rad, United States).
Detection of NETs with fluorescence microscopy
Neutrophils were seeded onto 0.01% poly-L-lysine coated coverslips and stimulated with DMEM medium collected from control or SARS-CoV-2 infected Vero cells (2 x 106 PFU/ml) and 100 nM phorbol 12-myristate 13-acetate (PMA) for 3 h at 37°C and 5% CO2. After stimulation, cells were fixed with 2% paraformaldehyde for 10 min and 0.05% Triton-X100 for 15 min followed by 1 h incubation in the blocking buffer consisting of 5% FBS in PBS. For citrullinated histone H3 staining, fixed neutrophils were incubated for 2 h with 0.5 µg/sample of primary anti-Histone H3 (citrulline R17) antibody (ab219407, Abcam, United Kingdom) followed by 1 h of a 0.5 µg/sample of secondary PE Donkey anti-rabbit IgG (minimal x-reactivity) Antibody (406421, Biolegend, United States). Coverslips were then washed with PBS and stained for DNA with 200 nM SYTOX™ Green Nucleic Acid Stain (Invitrogen, United States) for 15 min. Finally, coverslips were mounted with Fluorescent Mounting Medium (Trevigen, United States) and images were collected with an Axiolab 5 fluorescence microscope (Zeiss, Germany) using Filter Sets 43 and 44 (Zeiss, Germany).
Detection of NETs using live-cell imaging
Isolated neutrophils were counted and 2 × 104 cells/well were seeded on a 96-well tissue culture flat-bottom plate in 100 µL of phenol-red free Gibco™ RPMI 1640 medium (Paisley, Scotland, United Kingdom) with 10% FBS (PAN-Biotech, Aidenbach, Germany), and were stained with 1.25 μg/mL Hoechst 33342 (Merck, United States) and 200 nM SYTOX™ Green Nucleic Acid Stain (Invitrogen, United States). Neutrophils were then treated with 100 µL of isolated mitochondria (to a final concentration of 20 mitochondria particles/cell) and heat-inactivated media collected from control or SARS-CoV-2–infected Vero E6 cells (to a final MOI of 1) and imaged for 3 h on a Cytation 7 Cell Imaging Multi-Mode Reader (BioTek, United States) using DAPI (excitation = 377, emission = 447) and GFP (excitation = 469, emission = 525) LED cubes. NETs were considered to be >20 µm SYTOX™ Green-positive objects and the relative NET formation was calculated from the area under the curve (AUC) of NETs formed over time normalized to the number of Hoechst-positive cells.
Flow cytometry of mitochondria and DNA-containing particles
The quantity and size of DNA-associated microparticles stained with 200 nM SYTOX™ Green Nucleic Acid Stain (Invitrogen, United States) and cell-free mitochondria stained with 100 nM Mitoview™ (Biotium, United States) were analyzed with a DxFlex flow cytometer (Beckman Coulter, United States) using a combination of V-SSC-A and FITC-A channels with a dynamic gain of 100 and a threshold for detection set to V-SSC-A (1,400) and FITC-A (700). The particle size was calculated using a Flow Cytometry Sub-Micron Particle Size Reference Kit (Invitrogen™, United States).
Statistical analysis
T-tests and ANOVA analyses were performed using GraphPad Prism v8.00 for Windows (GraphPad Software, La Jolla, CA, United States). Data are presented as the mean and standard deviation (SD). p values < 0.05 were considered statistically significant.
Results
Infectious cell culture medium and uninfected cells–derived medium induce the formation of NETs
The induction of NETs by the medium containing the live SARS-CoV-2 virus was first analyzed with fluorescent microscopy. NETs were identified according to positive staining for histone H3 citrullination and a web-like morphology of the released DNA. Treatment with both an infectious medium containing SARS-CoV-2 (set to a MOI of 1) and an uninfected medium from Vero E6 cells induced the formation of NETs with comparable intensities, and promoted histone citrullination in the majority of the neutrophils. PMA, used as a positive control, induced substantial NETs formation while control, untreated neutrophils were negative for both histone H3 citrullination and NET release (Figure 1A).
Cell culture media derived from SARS-CoV-2–infected as well as uninfected cells contain extracellular mitochondria
The content of both the control and SARS-CoV-2–infected cell media was, after heat inactivation, analyzed using flow cytometry for the presence of damage-associated molecular patterns (DAMPs), represented by submicron particles associated with nucleic acids or mitochondrial components (Figure 1B). Heat inactivation of the media was performed in order to inactivate the SARS-CoV-2 virus. The concentration of nucleic acid–associated particles was ∼11 times higher in media collected from SARS-CoV-2–infected Vero E6 cells compared to the media from uninfected Vero E6 cells, with more than 90% of the particles forming a population with an estimated size of up to 150 nm (p < 0.01) (Figures 1B, C). When analyzed for the presence of mitochondrial components, both media from uninfected and SARS-CoV-2–infected Vero E6 cells contained a similar amount of particles sized between 120 and 500 nm (Figures 1B, D). The quantity of mtDNA was analyzed using qPCR, with no difference being observed in the mitochondrial DNA copy number between uninfected media from Vero E6 cells and media from Vero E6 cells infected with SARS-CoV-2 (Figure 1E).
Exogenous mitochondria induce NETs similarily to infectious and control media
Finally, NETs formation in response to exogenously administered mitochondria and heat-inactivated uninfected and SARS-CoV-2–infected Vero E6 cell media was analyzed using live-cell imaging (Figure 2A). Heat inactivation of the media was performed in order to inactivate the SARS-CoV-2 virus and to allow for the quantitative detection of NETs using live-cell microscopy. The concentration of extracellular mitochondria was set to 20 mitochondrial particles/cell, which is the average concentration of mitochondria found in both media collected from uninfected and SARS-CoV-2–infected Vero E6 cells. All of the samples induced NETs when compared to the control, and the intensity of the response did not differ between the treatments (p < 0.05) (Figure 2B).
Discussion
Since their discovery, the formation of NETs was found to be induced by a plethora of different stimuli, including viruses (Saitoh et al., 2012; Raftery et al., 2014; Funchal et al., 2015). At the beginning of the COVID-19 pandemic, several studies have shown that SARS-CoV-2 infection results in an aberrant neutrophil activation, with a subsequent release of NETs (Zuo et al., 2020; Guéant et al., 2021; Thierry, 2021). Herein, we have tested the capacity of both infectious and heat-inactivated media containing the SARS-CoV-2 virus and collected from Vero cells to induce NETs formation. We found that both media induce the formation of NETs and extracellular mitochondria that are released from damaged cells during virus cultivation largely mediate their induction.
Back in 2020, two research teams were fast to report that infectious and heat-inactivated SARS-CoV-2 can induce the formation of NETs, but that formaldehyde fixation inhibits their capacity to do so (Arcanjo et al., 2020; Veras et al., 2020). Veras and others have even proposed a mechanism where the virus stimulates NET release upon binding to neutrophil angiotensin converting enzyme (ACE2) (Veras et al., 2020). In both studies, SARS-CoV-2 induced the formation of NETs, even at very low concentrations, starting from an MOI of 0.5–1. This dose was found to be surprisingly lower than what is typically required for other pathogenic stimuli such as bacteria or fungi (Hoppenbrouwers et al., 2017).
Similar to these studies, we have also cultivated SARS-CoV-2 on an immortalized Vero E6 cell line that is standardly used as a substrate for virus isolation. Interestingly, we have observed that both media derived from SARS-CoV-2–infected Vero E6 cells and uninfected cells exhibited equal potency in inducing the formation of NETs. This suggests that other components of the media than just the virus itself contribute to neutrophil activation. The successful spread of a virus requires lysis of the infected cell, which, in the case of coronaviruses, results in significant destruction of pneumocytes (Darden et al., 2020). We have therefore hypothesized that mitochondria released from dead cells accumulated in cultivation media and were acting as DAMPs. On the other hand, cell lysis appears to be not primarily driven by SARS-CoV-2, as both infected and uninfected media contained a similar concentration of mitochondria. It may be possible that cytopathy was not as pronounced during media collection, and this can be considered a limitation of this study. Nevertheless, the titer of the collected virus and the multiplicity of infection used to induce NETs were similar to those in the studies by Arcanjo et al. (2020) and Veras et al. (2020); therefore, these studies and our work can be considered comparable.
Due to their evolutionary origin, mitochondria share several bacterial features, such as formylated peptides and unmethylated DNA, that have been described as potent NET inducers (Zhang et al., 2010; Itagaki et al., 2015). This would be in line with previous observations, where NETs were not found to be present during mild influenza virus infection, but have been shown to exacerbate the pathology of severe influenza pneumonitis characterized by lung damage (Narasaraju et al., 2011; Ellis et al., 2015; Pillai et al., 2016). It must be noted that during cell lysis, other intracellular components such as peroxisomes, ribosomes or fragments of nuclei might be released into the medium and can also act as DAMPs, although it was not yet verified whether they actually induce NETs as well. On the contrary, hantaviruses were reported to strongly stimulate NET release in infected patients, even without causing any cytopathic effect on endothelial or epithelial cells (Raftery et al., 2014). Induction of NETs by viruses may therefore be more complex; however, cell damage may at least augment this phenomenon. Indeed, our flow cytometry analysis revealed that media derived from both SARS-CoV-2–infected and uninfected Vero E6 cells contained a lot of mitochondria, which was also confirmed by qPCR. We have therefore stimulated neutrophils with isolated mitochondria set to an average dose found in the Vero E6 cell media and we observed a similar rate of NET induction. Nevertheless, while it appears that the actual virus contributes very little to NETs formation, it would be interesting to investigate its potency in inducing NETs when purified. This evaluation could determine whether the virus either contributes to or interferes with NETs formation induced by mitochondria. We propose that mitochondria released from dying cells during SARS-CoV-2 infection contribute to the aberrant activation of neutrophils, resulting in the formation of NETs. While this phenomenon warrants more extensive verification, we want to highlight the importance of the surrounding milieu when analyzing NET induction by viruses in vitro. In vivo, a virus is inevitably linked with its host cell, and this should be taken into account during experiment design. Understanding the mechanisms involved in NET induction during SARS-CoV-2 infection is thus vital not just for COVID-19 pathology, but for other viral infections as well.
Data availability statement
The raw data supporting the conclusion of this article will be made available by the authors, without undue reservation.
Ethics statement
The studies involving humans were approved by the Ethics Committee of the University Hospital Bratislava (workplace Ruzinov under number EK 218/2020). The studies were conducted in accordance with the local legislation and institutional requirements. The participants provided their written informed consent to participate in this study. Ethical approval was not required for the studies on animals in accordance with the local legislation and institutional requirements because only commercially available established cell lines were used.
Author contributions
JJ provided investigation, methodology, and drafting of the manuscript. MP provided investigation, methodology, data analysis and curation, project administration, and writing of the original draft. MS provided investigation and methodology. BK provided supervision, review, and editing of the manuscript. PC provided conceptualization, methodology, supervision, funding acquisition, project administration, and review and editing. All authors contributed to the article and approved the submitted version.
Funding
This work was supported by grants from the Slovak Research and Development Agency, Nos. PP-COVID-20-0016, PP-COVID-20-0017, and APVV-21-0378, as well as by the European Union’s Horizon 2020 Research and Innovation Program (EVA-GLOBAL project, grant agreement number 871029).
Conflict of interest
The authors declare that the research was conducted in the absence of any commercial or financial relationships that could be construed as a potential conflict of interest.
Footnotes
1Available at: https://www.european-virus-archive.com/virus/sars-cov-2-strain-slovakiask-bmc52020
References
Arcanjo, A., Logullo, J., Menezes, C. C. B., de Souza Carvalho Giangiarulo, T. C., Dos Reis, M. C., de Castro, G. M. M., et al. (2020). The emerging role of neutrophil extracellular traps in severe acute respiratory syndrome coronavirus 2 (COVID-19). Sci. Rep. 10 (1), 19630. doi:10.1038/s41598-020-76781-0
Auld, S. C., Caridi-Scheible, M., Blum, J. M., Robichaux, C., Kraft, C., Jacob, J. T., et al. (2020). ICU and ventilator mortality among critically ill adults with coronavirus disease 2019. Crit. Care Med. 48 (9), e799–e804. doi:10.1097/ccm.0000000000004457
Brinkmann, V., Reichard, U., Goosmann, C., Fauler, B., Uhlemann, Y., Weiss, D. S., et al. (2004). Neutrophil extracellular traps kill bacteria. Science 303 (5663), 1532–1535. doi:10.1126/science.1092385
Darden, D. B., Hawkins, R. B., Larson, S. D., Iovine, N. M., Prough, D. S., and Efron, P. A. (2020). The clinical presentation and immunology of viral pneumonia and implications for management of coronavirus disease 2019. Crit. Care Explor 2 (4), e0109. doi:10.1097/cce.0000000000000109
Ellis, G. T., Davidson, S., Crotta, S., Branzk, N., Papayannopoulos, V., and Wack, A. (2015). TRAIL+ monocytes and monocyte-related cells cause lung damage and thereby increase susceptibility to influenza-Streptococcus pneumoniae coinfection. EMBO Rep. 16 (9), 1203–1218. doi:10.15252/embr.201540473
Funchal, G. A., Jaeger, N., Czepielewski, R. S., Machado, M. S., Muraro, S. P., Stein, R. T., et al. (2015). Respiratory syncytial virus fusion protein promotes TLR-4-dependent neutrophil extracellular trap formation by human neutrophils. PLoS One 10 (4), e0124082. doi:10.1371/journal.pone.0124082
Guéant, J. L., Fromonot, J., Guéant-Rodriguez, R. M., Lacolley, P., Guieu, R., and Regnault, V. (2021). Blood myeloperoxidase-DNA, a biomarker of early response to SARS-CoV-2 infection? Allergy 76, 892–896. doi:10.1111/all.14533
Hoppenbrouwers, T., Autar, A. S. A., Sultan, A. R., Abraham, T. E., van Cappellen, W. A., Houtsmuller, A. B., et al. (2017). In vitro induction of NETosis: comprehensive live imaging comparison and systematic review. PLoS One 12 (5), e0176472. doi:10.1371/journal.pone.0176472
Itagaki, K., Kaczmarek, E., Lee, Y. T., Tang, I. T., Isal, B., Adibnia, Y., et al. (2015). Mitochondrial DNA released by trauma induces neutrophil extracellular traps. PLoS One 10 (3), e0120549. doi:10.1371/journal.pone.0120549
Klok, F. A., Kruip, M., van der Meer, N. J. M., Arbous, M. S., Gommers, D., Kant, K. M., et al. (2020). Incidence of thrombotic complications in critically ill ICU patients with COVID-19. Thromb. Res. 191, 145–147. doi:10.1016/j.thromres.2020.04.013
Kovacech, B., Fialova, L., Filipcik, P., Skrabana, R., Zilkova, M., Paulenka-Ivanovova, N., et al. (2022). Monoclonal antibodies targeting two immunodominant epitopes on the spike protein neutralize emerging SARS-CoV-2 variants of concern. EBioMedicine 76, 103818. doi:10.1016/j.ebiom.2022.103818
Lagunas-Rangel, F. A. (2020). Neutrophil-to-lymphocyte ratio and lymphocyte-to-C-reactive protein ratio in patients with severe coronavirus disease 2019 (COVID-19): A meta-analysis. J. Med. Virol. 92, 1733–1734. doi:10.1002/jmv.25819
Narasaraju, T., Yang, E., Samy, R. P., Ng, H. H., Poh, W. P., Liew, A. A., et al. (2011). Excessive neutrophils and neutrophil extracellular traps contribute to acute lung injury of influenza pneumonitis. Am. J. Pathol. 179 (1), 199–210. doi:10.1016/j.ajpath.2011.03.013
Papayannopoulos, V. (2018). Neutrophil extracellular traps in immunity and disease. Nat. Rev. Immunol. 18 (2), 134–147. doi:10.1038/nri.2017.105
Pillai, P. S., Molony, R. D., Martinod, K., Dong, H., Pang, I. K., Tal, M. C., et al. (2016). Mx1 reveals innate pathways to antiviral resistance and lethal influenza disease. Science 352 (6284), 463–466. doi:10.1126/science.aaf3926
Raftery, M. J., Lalwani, P., Krautkrӓmer, E., Peters, T., Scharffetter-Kochanek, K., Krüger, R., et al. (2014). β2 integrin mediates hantavirus-induced release of neutrophil extracellular traps. J. Exp. Med. 211 (7), 2055OIA105–97. doi:10.1083/jcb.2055oia105
Saitoh, T., Komano, J., Saitoh, Y., Misawa, T., Takahama, M., Kozaki, T., et al. (2012). Neutrophil extracellular traps mediate a host defense response to human immunodeficiency virus-1. Cell Host Microbe 12 (1), 109–116. doi:10.1016/j.chom.2012.05.015
Thierry, A. R. (2021). Does the newly observed inflammatory syndrome in children demonstrate a link between uncontrolled neutrophil extracellular traps formation and COVID-19? Pediatr. Res. 89, 716–717. doi:10.1038/s41390-020-0996-1
Veras, F. P., Pontelli, M. C., Silva, C. M., Toller-Kawahisa, J. E., de Lima, M., Nascimento, D. C., et al. (2020). SARS-CoV-2-triggered neutrophil extracellular traps mediate COVID-19 pathology. J. Exp. Med. 217 (12), e20201129. doi:10.1084/jem.20201129
Zhang, Q., Raoof, M., Chen, Y., Sumi, Y., Sursal, T., Junger, W., et al. (2010). Circulating mitochondrial DAMPs cause inflammatory responses to injury. Nature 464 (7285), 104–107. doi:10.1038/nature08780
Keywords: neutrophil extracellular traps, SARS-CoV-2, mitochondria, neutrophil, DAMPs
Citation: Janko J, Sláviková M, Klempa B, Celec P and Pastorek M (2023) In vitro induction of neutrophil extracellular traps by SARS-CoV-2 is biased by extracellular mitochondria. Acta Virol. 67:11801. doi: 10.3389/av.2023.11801
Received: 13 July 2023; Accepted: 17 August 2023;
Published: 01 September 2023.
Edited by:
Katarina Polcicova, Slovak Academy of Sciences, SlovakiaReviewed by:
Tatiana Betakova, Comenius University, SlovakiaIvan Kosik, National Institute of Allergy and Infectious Diseases (NIH), United States
Copyright © 2023 Janko, Sláviková, Klempa, Celec and Pastorek. This is an open-access article distributed under the terms of the Creative Commons Attribution License (CC BY). The use, distribution or reproduction in other forums is permitted, provided the original author(s) and the copyright owner(s) are credited and that the original publication in this journal is cited, in accordance with accepted academic practice. No use, distribution or reproduction is permitted which does not comply with these terms.
*Correspondence: Michal Pastorek, michal.pastorek@fmed.uniba.sk