Deconstructing motor and non-motor aspects of dystonia with neuroimaging
- Rush Parkinson’s Disease and Movement Disorders Program, Rush University Medical Center, Chicago, IL, United States
Dystonia, the third most common movement disorder, is clinically characterized by involuntary muscle contractions leading to abnormal, patterned movements and postures that are often activated or worsened by initiation of movement. In addition to motor features, the presence and contribution of non-motor features including sensory and psychiatric features is increasingly recognized. However, the underlying pathophysiology behind dystonia and its fascinating motor and non-motor presentations remains inadequately understood. Advances in neuroimaging may hold the key. This review outlines brain imaging studies, with an intentional focus on our work, conducted using different structural and functional neuroimaging modalities, focused on dystonia and its motor and non-motor clinical presentations. It highlights the different parts of the human brain that may be implicated with these aspects of this network disorder. Finally, current limitations and promising future directions to deconstruct this knot and take a leap forward are mentioned.
Introduction
The earliest recorded documentation of dystonia is thought to be the abnormal neck positions in the tomb of Bishop Pedro de Osma in the Cathedral of Burgo de Osma in Spain. These carvings were executed in 1258 [1]. A few centuries later, Dr. Charcot coined the term “nevroses” for conditions without an identifiable neuroanatomical cause in 1888. In 1911, Dr. Oppenheim introduced the term “dystonia” as a unifying descriptor of the condition. For a few years thereafter, our understanding of dystonia was that these movements were unconscious conflicts transduced into physical symptoms. This psychoanalytical model popularized by Dr. Sigmund Freud describes, at least partially, our understanding of functional neurological disease and may be influenced by curious, inadequately understood aspects of dystonia such as sensory tricks. Subsequently, Dr. David Marsden’s clinical and electrophysiological research in the 1990s on adult-onset focal dystonias established a physical basis for dystonia [1]. In 1991, a detailed published description of 300 patients with dystonia in the clinic highlighted that cervical dystonia (CD) was the most common dystonia (86%). The onset of CD was most common between the 4th and 6th decade of life. Pain was reported in nearly 70% and psychiatric disorders in nearly 20% of patients [2].
The primary criteria of our current definition of dystonia is based on motor features only: “Cervical dystonia is characterized by sustained or intermittent neck movements caused by involuntary muscle contractions, resulting in abnormal movements and postures of head, neck, and/or shoulders. Movements are often patterned and tremor may be present.” [3] However, non-motor features including sensory trick and pain have been recognized as supportive criteria instrumental for diagnosis [3]. In addition, it is now well established that dystonia may additionally present with psychiatric or even cognitive manifestations [4]. Monogenic dystonias vary widely in topography, age of onset, spread, and severity of motor and non-motor features [5]. Dystonia may not uncommonly present as a feature with other neurological disorders such as Parkinson’s disease, Corticobasal syndrome, or Multiple-System Atrophy. With such heterogeneity of presentation, this rabbit hole keeps on getting deeper.
Many centuries after it was presumably noted and over a century after the term “dystonia” was coined, the underlying pathophysiology behind dystonia and its fascinating motor and non-motor presentations remains inadequately understood. Advances in neuroimaging may hold the key.
Insights into pathophysiology of dystonia
A short primer on magnetoencephalography (MEG)
The brain consists of populations of neurons that work together to send signals to other parts of the brain. These brain networks consist of voltage-gated ion channels that drive action potentials and spiking membrane potentials. The oscillatory activity thus created contributes to synchronous activity in neighboring neurons. Such synchronous activity, when it involves a large population of neurons, leads to electrical field oscillations. The magnetic field produced by these oscillations is detected by MEG [6, 7]. A metric used to quantify the frequency and amplitude of this synchronicity of neuronal patterns is coherence. MEG offers a direct representation of connectivity as it measures intra-cellular current. MEG fields pass through the head without distortion [8]. Data capture through MEG requires the patient to lie relatively still. Movement may worsen environmental magnetic interference. This can be challenging. However, given its good spatial and excellent temporal resolution, it is uniquely placed in the study of movement disorders [6, 9, 10] (Figure 1).
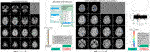
FIGURE 1. Representative MEG scan data from two different studies. The image on the left shows involvement of frontal and parietal regions during a Continuous Performance Task when the subject is deciding to press a button or inhibit the desire to press a button. The image on the right represents regions of mean coherence pre- and post-sensory trick.
MEG, resting state connectivity and treatment in dystonia
Using MEG, we sought to identify resting-state connectivity that distinguishes CD from healthy controls [11]. We identified 5 CD patients and 5 age- and sex-matched controls. All subjects underwent MRIs of the brain. MEG scans were obtained with results displayed on the subject’s MRI with images co-registered to the subject’s digitized head shape at the time of data collection. The coherence was calculated between all pairings (54 regions, 1431 locations in both hemispheres) of active cortical locations. For each active source, pairwise coherence across frequencies for all active cortical locations was calculated and only significant coherence values with a large effect size were considered. Results showed highly coherent networks in the cortico-striatal regions (fronto-striatal, occipito-striatal, parieto-striatal and temporo-striatal) in controls as compared to CD patients, which may represent an underlying aberrant mechanism in CD [11]. To assess the effect of botulinum toxin on CD, we additionally captured MEG data in CD subjects pre- and post-botulinum toxin injections [11]. Results showed an increase in coherence overall in the afore-mentioned cortico-striatal pathways in patients after botulinum toxin injections. In addition, a new pathway showed significantly increased coherence: the occipito-temporal pathway. After applying Benjamini-Hochberg correction to reduce false discovery rate and further improve specificity, this increased coherence localized to a connection between the left Putamen and right Superior Parietal gyrus. While the basal ganglia are central to motor function, the superior parietal lobule has been noted to be important for sensorimotor integration by maintaining an internal representation of the body’s state [12]. As such these results highlighted the role of sensorimotor integration with clinical benefit seen with botulinum toxin in CD. It further supports a “central” effect of toxin secondary to sensory feedback. Finally, with an increase in coherence appreciated within and between hemispheres, these data highlighted the importance of local and global networks in CD [11].
Other imaging modalities have been used to study network abnormalities in dystonia. Aberrant fronto-striatal connectivity and cerebellar involvement has been reported using FDG-PET and DTI [13], altered connectivity within the sensorimotor, executive control, and the primary visual networks using fMRI [14], and motor-related altered activity in the primary somatosensory cortex, cerebellum, dorsal premotor and posterior parietal cortices, and occipital cortex using fMRI in another study [15]. Using lesion network mapping, authors reported that a positive connectivity to the cerebellum and negative connectivity to the somatosensory cortex distinguished CD compared to lesions causing other neurological symptoms. They concluded that this connectivity signature was a specific marker for CD [16]. In 2019, the effect of botulinum toxin in CD at 6 months was assessed using 3T fMRI. The authors reported altered sensorimotor integration with involvement of the basal ganglia, the thalamus, and the sensorimotor cortex, with a “shift towards normal brain function” in CD patients especially in the motor cortex and these regions [17].
In addition to CD, MEG has been used to investigate other forms of dystonia. These studies further highlight the role of the sensorimotor cortex in dystonia. In their study on the effect of successful rehabilitation on 11 subjects with Writer’s cramp and 10 controls, authors reported “normalization” of the MEG somatosensory map of the cortex with hand representation similar to healthy controls and significantly different from untreated Writer’s cramp [18]. In another study on Writer’s cramp using diffusion-weighted MRI for tractography and fMRI during a finger tapping task, authors reported a possible aberrant downstream influence of parietal multimodal sensory association region on fine motor control network that could be “artificially restored” with therapy [19]. Our MEG study of a case of Embouchure dystonia revealed increased overall coherence in bilateral parietal and inferior frontal lobes, with increased gamma frequency while playing the instrument. This finding was thought to reflect reduced intracortical inhibition and abnormal sensorimotor processing [20]. Finally, the degree of pallido-cerebellar coupling has been showed to inversely correlate with motor symptom severity in idiopathic dystonia [21].
Head and neck tremor in CD
Unlike with other phenomenology, dystonia accompanied by tremor is considered an “isolated” dystonia [22]. Tremor and dystonia, especially of the head and neck region, are commonly seen together [23]. Head tremor is recognized as an early potential manifestation of CD. Such an irregular, jerky tremor of the head and neck with a null-point may be seen as a manifestation of acute or chronic cerebellar dysfunction [24–27]. An anecdotal observation at the University of Cincinnati was that CD patients with a head tremor at onset of CD may experience difficulty with gait and balance over time. This presentation is distinct from other CD patients who had a more “tonic” or non-tremulous presentation.
To further delineate differences between such patients with a tremor dominant (head tremor at onset of CD) and non-tremor dominant CD, a multi-center effort sought to study data captured through the Dystonia Coalition [28]. Patients with tremor dominant CD were noted to be older, with a longer disease duration and more likely to be female. Separately, blinded assessors rated videos of patients with tremor dominant CD and (age, sex, and disease duration) matched non-tremor dominant CD patients using validated scales of dystonia and ataxia severity. Tremor dominant CD patients had greater ataxia and milder dystonia [28]. Additionally, speech, gait, postural ataxia, and appendicular tremor were worse in tremor dominant CD patients. The authors concluded that CD patients with head tremor at onset may be an “ataxic-subset” of CD, which becomes apparent after years of progression [28]. To further explore the role of the cerebellum in this distinct CD subset, we proceeded with a study of eye movements to assess motor adaptation in tremor dominant CD patients and healthy controls [29]. Disorders of the inferior olive and its connections to the cerebellum have been implicated in Impaired motor adaptation and resultant dysmetria [30]. We found that tremor dominant CD patients were unable to increase saccade gain by 25% in both fast and slow timescales thereby suggesting a maladaptive cerebellar outflow disorder [29].
Structural imaging of the cerebellum in cervical dystonia and tremor
With data from epidemiology and eye physiology of tremor and dystonia indicative of a diseased cerebellum, we sought to utilize structural imaging of the cerebellum to corroborate these findings [31]. Patients with tremor dominant CD and age- and sex-matched non-tremor dominant CD patients underwent MRI of the brain. Additionally, patients underwent gait mapping and assessment using validated scales. Our study found that tremor dominant CD patients exhibited greater atrophy of the superior vermis, greater median gait variability (a sign of a “cerebellar” gait), and worse disability on the ataxia scale. As such, it reiterated the role of the cerebellum in a distinct clinical subtype of CD [31]. These data could be helpful in clinical prognostication and clinical trial inclusion.
The cerebellum has emerged as a region of great interest in dystonia with potential contributions to motor and non-motor features [32]. Rapid advances in neuroimaging and neurophysiology of the cerebellum will be key to fill our gaps in knowledge of physiology and pathophysiology [33–35]. As the field of cerebellar stimulation continues to mature, these data could have a direct impact on therapeutics [36].
Non-motor aspects of dystonia
Cognition
The topic of cognition in dystonia has garnered relatively low academic interest, in part due to it not being the primary symptom patients present with in clinic. Cognitive assessment can be challenging in dystonia, as can be the conclusions that may be drawn from neuropsychological testing [37]. There are a number of pertinent factors that may influence conclusions: age, pre-morbid cognitive function, type of dystonia, pain, psychiatric symptoms including anxiety, and anti-cholinergic medication burden (not uncommonly used for treatment in dystonia). Unlike other movement disorders, the presence of executive dysfunction in dystonia has been debated over the years [38–40]. Tests used to measure executive function may depend on time to complete a task as a metric, and this may introduce a motor confound in patients with movement disorders; [37]. Tests may not be sensitive enough to differentiate neurodegenerative executive dysfunction from normative age-related changes. Finally, our conclusions may be gleaned from large epidemiological studies where screening cognitive measures (such as Mini mental status exam or Montreal cognitive assessment) are used to comment on the robustness of different cognitive domains [37]. The resulting heterogeneity of tests makes it challenging to decisively comment on cognition in movement disorders, especially dystonia [41].
Assessing executive function in CD using MEG
To assess executive function in CD and the effect of BoNT on the same, we captured MEG data on subjects with CD and age- and sex-matched controls while they performed a continuous performance task [42]. As a part of this task, subjects underwent 100 trials of random letters being shown on a screen briefly for 150 ms with a 1.8 s inter-stimulus interval. Whenever they saw the letter “A” followed by the letter “X,” they were instructed to press a button as quickly as possible. Subjects were asked to complete this task before and after BoNT. Only those with documented robust clinical response from prior BoNT injections were included in the study. Compared to controls, subjects demonstrated greater number of errors despite taking a longer time to make decision, pre-BoNT [42]. After BoNT, both the time to make decisions and number of errors improved, albeit still worse than controls. During performance of this task, subjects demonstrated greater coherence compared to controls in the cortico-frontal (fronto-frontal, fronto-parietal, fronto-temporal, fronto-striatal, fronto-occipital) pathways and the parieto-parietal, parieto-striatal and temporo-parietal pathways. Comparing subjects post versus pre-BoNT, the following pathways were significantly different: fronto-parietal, fronto-cingulate, fronto-occipital, parieto-insular and parieto-temporal [42]. Our pilot study highlighted the impairment of attention/executive function and associated networks in CD. It further reiterated the role of frontal networks in attention/executive function and the parietal lobe in selective attention/preferential processing [43]. The mechanism of benefit seen in cognition is unclear and was not investigated in our study. While some have speculated a direct benefit of toxin on non-motor features including cognition, it is likely that at least, a substantial part of the improvement can be attributed to improvement in motor symptoms and pain, which improves attention [44].
Sensory trick/alleviating maneuver
Sensory trick or alleviating maneuver refers to a fascinating, characteristic feature in CD where commonly, touching a body segment ameliorates the dystonia posture or abnormal movement [45]. It has now been recognized as a supportive diagnostic criteria for isolated CD [3]. It has been proposed that this maneuver acts via influencing sensorimotor integration, by enhancing pathways between the occipital and parietal lobes through proprioception.
Neuroimaging and sensory trick in CD
Using MEG, we studied cerebral oscillations in a patient with CD and an effective sensory trick [42]. Four scans were collected: resting state, with sensory trick, before BoNT, and after BoNT. Areas in the inferior frontal, left cerebellum and left parietal were noted to be active before sensory trick, which changed to areas in the occipital and left temporal lobe after the sensory trick. There was a suggestion of increase in Gamma (>25 Hz) activity with a decrease in Alpha (8–12 Hz) activity with the sensory trick. Moreover, change in coherence with sensory trick and BoNT indicated that they may share similar pathways to clinical benefit, specifically occipital and temporal lobe [42].
Subsequently, a study sought to investigate sensory trick using functional MRI. CD patients without a sensory trick were found to have increased functional connectivity of sensorimotor network relative to controls, while CD patients with an effective sensory trick demonstrated a decrease in sensorimotor network connectivity. Finally, imagination of the sensory trick by CD subjects showed increased recruitment of the cerebellum bilaterally, suggesting its role in modulating cortical activity [46]. More recently, a study of sensory trick using EEG and EMG demonstrated alpha desynchronization in the sensorimotor cortex and theta desynchronization in the sensorimotor and posterior parietal cortex with an effective sensory trick. Greater decrease in muscle activity correlated with higher desynchronization in the alpha and theta bands in the sensorimotor and posterior parietal areas [47].
The therapeutic effect of an effective sensory trick is well established but is yet to be consistently harnessed as a treatment modality. In future efforts, quantification of the therapeutic benefit seen with sensory trick will greatly help in its study in clinical trials.
While the majority of neuroimaging studies have focused on disorder of motor function in dystonia, it is imperative that future efforts focus on non-motor features including clinically-relevant ones such as anxiety [48]. Understanding these networks would move the needle in understanding whether these are intrinsic to dystonia or a consequence of it. As with other movement disorders, a therapeutic approach towards motor disability that under-estimates anxiety would likely be sub-optimal.
Localization in dystonia and the neural integrator model
The field of neurology has traditionally been based on localization of specific symptoms to singular brain regions. Through neuroimaging, specific motor and non-motor symptoms in dystonia have been localized to different regions of the brain, albeit with some overlap. Based on afore-mentioned data, the sensorimotor cortex has been implicated in the pathophysiology and response to botulinum toxin. The cerebellum appears to drive head and neck tremor in CD. The frontal lobe and its connection with the striatum is involved with cognitive tasks. As expected from a disorder of movement, the basal ganglia present as a culprit. In addition, they may be the generator of anxiety though that needs further assessment. As such, dystonia is truly a network disorder with the strength of connectivity between regions representing the severity of associated clinical manifestations.
An interesting hypothesis that may explain widespread involvement of networks in CD is the neural integrator model [49, 50]. This model has two key aspects: One, the basal ganglia, cerebellum and neck muscles (through proprioception) provide feedback to an integrator loop to prevent drifts in the position of head and neck. Second, disturbance anywhere in this feedback network may change the entire network [49, 50]. This conceptual model would also explain how botulinum toxin (through feedback through the neck muscles) and stimulation of the basal ganglia can both lead to clinical improvement in CD [49, 50].
Limitations and future directions: gaps, pitfalls and promises
The primary limitation of literature in structural and functional neuroimaging, including that in dystonia, is the absence of a satisfactory sample size to adequately power studies. As a result, false positive results are aplenty, and replicability suffers [51]. Combining clinical data with pre-specified regions of interest may help address this limitation. Neuroimaging in dystonia suffers from the chicken or egg conundrum, and cause versus effect/compensation is tough to comprehend [52] (Figure 2). An extension of this existential question lies in studying differences in brain structure and function in isolated dystonia versus dystonia as a manifestation of neurological entities (such as corticobasal syndrome). As dystonia may be initiated or worsened by voluntary action, modalities with higher temporal resolution may help shed light on this problem. The advent of 7-Tesla (and now, 14-Tesla) magnets will improve visualization of smaller structures with greater spatial resolution and reduce noise. In addition, a possibly shorter scan time may presumably improve our study sample size [53, 54]. We have barely scratched the surface of appropriate application of machine learning in identifying patterns of presentation, response to treatments and overcoming the problem of a low sample size [55]. Molecular brain imaging, not covered in this review, continues to be a promising tool to question the neural basis of dystonia and to identify targets for therapeutics in humans [56, 57]. Future considerations in neuroimaging must include the effect of environment and genetics on the study of brain organization through structural and functional neuroimaging in dystonia [58]. An approach that connects the dots between genes/proteins, biochemistry, structures and networks and clinical phenotypes will perhaps be our best bet to deconstruct this knot and take a substantial leap forward [59].
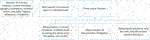
FIGURE 2. The “chicken or the egg” conundrum in the motor pathophysiology of dystonia. Based on neurophysiology and imaging studies, a number of regions within the CNS have been proposed to cause dystonia. Some are non-specific to dystonia. It is unclear whether these results represent an effect of dystonia or the pathophysiology behind the clinical manifestation of dystonia. As such, these show correlation and do not offer evidence of causation. Three major themes have been proposed [52].
Author contributions
AM was responsible for writing the first draft and revision of the final draft.
Conflict of interest
The author declares that the research was conducted in the absence of any commercial or financial relationships that could be construed as a potential conflict of interest.
Acknowledgments
I would like to acknowledge my mentors and colleagues without whom the highlighted work could never be done (not an exhaustive list, in no particular order): Christos Sidiropolous, Susan Bowyer, Andrew Zillgitt, Abdullah Alshammaa, Alberto Espay, Aasef Shaikh, Christopher Goetz, Glenn Stebbins, Dana Sugar, Deepal Shah-Zamora and Cynthia Comella. I especially thank Bowyer for her help with Figure 1. Finally, I thank the Dystonia Medical Research Foundation for the 2019 Clinical fellowship award towards our work on head tremor and the cerebellum in cervical dystonia. The text highlights some of our work on head tremor in cervical dystonia which was supported in part by a grant to the Dystonia Coalition (U54 TR001456 and NS065701) from the Office of Rare Diseases Research (ORDR) in the National Center for Advancing Translational Sciences (NCATS) and the National Institute of Neurological Disorders and Stroke (NINDS). The sponsors had no role in the design and conduct of the study; collection, management, analysis, and interpretation of the data; preparation, review, or approval of the manuscript; and decision to submit the manuscript for publication.
References
1. Newby, RE, Thorpe, DE, Kempster, PA, and Alty, JE. A history of dystonia: Ancient to modern. Mov Disord Clin Pract (2017) 4(4):478–85. doi:10.1002/mdc3.12493
2. Jankovic, J, Leder, S, Warner, D, and Schwartz, K. Cervical dystonia: Clinical findings and associated movement disorders. Neurology (1991) 41(7):1088–91. doi:10.1212/wnl.41.7.1088
3. Albanese, A, and Bhatia, KP. Isolated cervical dystonia: Diagnosis and classification. Mov Disord (2023). doi:10.1002/mds.29387
4. Balint, B, Mencacci, NE, Valente, EM, Pisani, A, Rothwell, J, Jankovic, J, et al. Dystonia. Nat Rev Dis Primers (2018) 4(1):25. doi:10.1038/s41572-018-0023-6
5. Sturchio, A, Marsili, L, Mahajan, A, Grimberg, MB, Kauffman, MA, and Espay, AJ. How have advances in genetic technology modified movement disorder nosology? Eur J Neurol (2020) 27(8):1461–70. doi:10.1111/ene.14294
6. Bowyer, SM. Coherence a measure of the brain networks: Past and present. Neuropsychiatr Electrophysiol (2016) 2(1):1. doi:10.1186/s40810-015-0015-7
7. Proudfoot, M, Woolrich, MW, Nobre, AC, and Turner, MR. Magnetoencephalography. Pract Neurol (2014) 14(5):336–43. doi:10.1136/practneurol-2013-000768
8. Singh, SP. Magnetoencephalography: Basic principles. Ann Indian Acad Neurol (2014) 17(Suppl. 1):S107–112. doi:10.4103/0972-2327.128676
9. Baillet, S. Magnetoencephalography for brain electrophysiology and imaging. Nat Neurosci (2017) 20(3):327–39. doi:10.1038/nn.4504
10. Marzetti, L, Basti, A, Chella, F, D'Andrea, A, Syrjälä, J, and Pizzella, V. Brain functional connectivity through phase coupling of neuronal oscillations: A perspective from magnetoencephalography. Front Neurosci (2019) 13:964. doi:10.3389/fnins.2019.00964
11. Mahajan, A, Alshammaa, A, Zillgitt, A, Bowyer, SM, LeWitt, P, Kaminski, P, et al. The effect of botulinum toxin on network connectivity in cervical dystonia: Lessons from magnetoencephalography. Tremor Other Hyperkinet Mov (N.Y.) (2017) 7:502. doi:10.7916/D84M9H4W
12. Wolpert, DM, Goodbody, SJ, and Husain, M. Maintaining internal representations: The role of the human superior parietal lobe. Nat Neurosci (1998) 1(6):529–33. doi:10.1038/2245
13. Carbon, M, Argyelan, M, and Eidelberg, D. Functional imaging in hereditary dystonia. Eur J Neurol. 2010;17 Suppl 1(0 1):58–64. doi:10.1111/j.1468-1331.2010.03054.x
14. Delnooz, CC, Pasman, JW, Beckmann, CF, and van de Warrenburg, BP. Task-free functional MRI in cervical dystonia reveals multi-network changes that partially normalize with botulinum toxin. PloS one (2013) 8(5):e62877. doi:10.1371/journal.pone.0062877
15. Burciu, RG, Hess, CW, Coombes, SA, Ofori, E, Shukla, P, Chung, JW, et al. Functional activity of the sensorimotor cortex and cerebellum relates to cervical dystonia symptoms. Hum Brain Mapp (2017) 38(9):4563–73. doi:10.1002/hbm.23684
16. Corp, DT, Joutsa, J, Darby, RR, Delnooz, CCS, van de Warrenburg, BPC, Cooke, D, et al. Network localization of cervical dystonia based on causal brain lesions. Brain (2019) 142(6):1660–74. doi:10.1093/brain/awz112
17. Brodoehl, S, Wagner, F, Prell, T, Klingner, C, Witte, OW, and Günther, A. Cause or effect: Altered brain and network activity in cervical dystonia is partially normalized by botulinum toxin treatment. Neuroimage Clin (2019) 22:101792. doi:10.1016/j.nicl.2019.101792
18. Bleton, JP, Vidailhet, M, Bourdain, F, Ducorps, A, Schwartz, D, Delmaire, C, et al. Somatosensory cortical remodelling after rehabilitation and clinical benefit of in writer's cramp. J Neurol Neurosurg Psychiatry (2011) 82(5):574–7. doi:10.1136/jnnp.2009.192476
19. Merchant, SHI, Frangos, E, Parker, J, Bradson, M, Wu, T, Vial-Undurraga, F, et al. The role of the inferior parietal lobule in writer's cramp. Brain (2020) 143(6):1766–79. doi:10.1093/brain/awaa138
20. Bulica, B, Sidiropoulos, C, Mahajan, A, Zillgitt, A, Kaminski, P, and Bowyer, SM. Sensorimotor integration and GABA-ergic activity in embouchure dystonia: An assessment with magnetoencephalography. Tremor Other Hyperkinet Mov (N.Y.) (2019) 9. doi:10.5334/tohm.487
21. Neumann, WJ, Jha, A, Bock, A, Huebl, J, Horn, A, Schneider, GH, et al. Cortico-pallidal oscillatory connectivity in patients with dystonia. Brain (2015) 138(Pt 7):1894–906. doi:10.1093/brain/awv109
22. Albanese, A, Bhatia, K, Bressman, SB, Delong, MR, Fahn, S, Fung, VSC, et al. Phenomenology and classification of dystonia: A consensus update. Mov Disord : Off J Mov Disord Soc (2013) 28(7):863–73. doi:10.1002/mds.25475
23. Shaikh, AG, Beylergil, SB, Scorr, L, Kilic-Berkmen, G, Freeman, A, Klein, C, et al. Dystonia and tremor: A cross-sectional study of the dystonia coalition cohort. Neurology (2021) 96(4):e563–e574. doi:10.1212/WNL.0000000000011049
24. Fahn, S. The varied clinical expressions of dystonia. Neurol Clin (1984) 2(3):541–54. doi:10.1016/s0733-8619(18)31090-9
25. Finsterer, J, Muellbacher, W, and Mamoli, B. Yes/yes head tremor without appendicular tremor after bilateral cerebellar infarction. J Neurol Sci (1996) 139(2):242–5. doi:10.1016/0022-510x(96)00153-0
26. Chansakul, C, Moguel-Cobos, G, and Bomprezzi, R. Head tremor secondary to MS resolved with rituximab. Neurol Sci : Off J Ital Neurol Soc Ital Soc Clin Neurophysiol (2011) 32(6):1157–60. doi:10.1007/s10072-011-0600-x
27. Rivest, J, and Marsden, CD. Trunk and head tremor as isolated manifestations of dystonia. Mov Disord : Off J Mov Disord Soc (1990) 5(1):60–5. doi:10.1002/mds.870050115
28. Merola, A, Dwivedi, AK, Shaikh, AG, Tareen, TK, Da Prat, GA, Kauffman, MA, et al. Head tremor at disease onset: An ataxic phenotype of cervical dystonia. J Neurol (2019) 266(8):1844–51. doi:10.1007/s00415-019-09341-w
29. Mahajan, A, Gupta, P, Jacobs, J, Marsili, L, Sturchio, A, Jinnah, HA, et al. Impaired saccade adaptation in tremor-dominant cervical dystonia-evidence for maladaptive cerebellum. Cerebellum (2021) 20(5):678–86. doi:10.1007/s12311-020-01104-y
30. Shaikh, AG, Wong, AL, Optican, LM, and Zee, DS. Impaired motor learning in a disorder of the inferior olive: Is the cerebellum confused? Cerebellum (London, England) (2017) 16(1):158–67. doi:10.1007/s12311-016-0785-x
31. Mahajan, A, Schroder, L, Rekhtman, A, Dwivedi, AK, Wang, LL, and Espay, AJ. Tremor-dominant cervical dystonia: A cerebellar syndrome. Cerebellum (London, England) (2021) 20(2):300–5. doi:10.1007/s12311-020-01211-w
32. Bologna, M, and Berardelli, A. The cerebellum and dystonia. In: Handbook of clinical neurology, 155. Elseveir (2018). p. 259–72. doi:10.1016/B978-0-444-64189-2.00017-2
33. Kumar, A, Lin, CC, Kuo, SH, and Pan, MK. Physiological recordings of the cerebellum in movement disorders. Cerebellum (2022) [Epub ahead of print]. doi:10.1007/s12311-022-01473-6
34. Diedrichsen, J, Verstynen, T, Schlerf, J, and Wiestler, T. Advances in functional imaging of the human cerebellum. Curr Opin Neurol (2010) 23(4):382–7. doi:10.1097/WCO.0b013e32833be837
35. Andersen, LM, Jerbi, K, and Dalal, SS. Can EEG and MEG detect signals from the human cerebellum? NeuroImage (2020) 215:116817. doi:10.1016/j.neuroimage.2020.116817
36. Tai, CH, and Tseng, SH. Cerebellar deep brain stimulation for movement disorders. Neurobiol Dis (2022) 175:105899. doi:10.1016/j.nbd.2022.105899
37. Mahajan, A, Deal, JA, and Carlson, M. Interventions in Parkinson's disease: Role of executive function. Front Biosci (Landmark edition) (2017) 22(3):416–27. doi:10.2741/4492
38. Jahanshahi, M, Rowe, J, and Fuller, R. Cognitive executive function in dystonia. Mov Disord : Off J Mov Disord Soc (2003) 18(12):1470–81. doi:10.1002/mds.10595
39. Bastos, MSC, Nickel, R, Camargo, CHF, and Teive, HAG. Patients with cervical dystonia demonstrated decreased cognitive abilities and visual planning compared to controls. Mov Disord Clin Pract (2021) 8(6):904–10. doi:10.1002/mdc3.13259
40. Romano, R, Bertolino, A, Gigante, A, Martino, D, Livrea, P, and Defazio, G. Impaired cognitive functions in adult-onset primary cranial cervical dystonia. Parkinsonism Relat Disord (2014) 20(2):162–5. doi:10.1016/j.parkreldis.2013.10.008
41. Kuyper, DJ, Parra, V, Aerts, S, Okun, MS, and Kluger, BM. Nonmotor manifestations of dystonia: A systematic review. Mov Disord : Off J Mov Disord Soc (2011) 26(7):1206–17. doi:10.1002/mds.23709
42. Mahajan, A, Zillgitt, A, Alshammaa, A, Patel, N, Sidiropoulos, C, LeWitt, PA, et al. Cervical dystonia and executive function: A pilot magnetoencephalography study. Brain Sci (2018) 8(9):159. doi:10.3390/brainsci8090159
43. Behrmann, M, Geng, JJ, and Shomstein, S. Parietal cortex and attention. Curr Opin Neurobiol (2004) 14(2):212–7. doi:10.1016/j.conb.2004.03.012
44. Allam, N, Frank, JE, Pereira, C, and Tomaz, C. Sustained attention in cranial dystonia patients treated with botulinum toxin. Acta Neurol Scand (2007) 116(3):196–200. doi:10.1111/j.1600-0404.2007.00862.x
45. Patel, N, Hanfelt, J, Marsh, L, and Jankovic, Jmembers of the Dystonia Coalition. Alleviating manoeuvres (sensory tricks) in cervical dystonia. J Neurol Neurosurg Psychiatry (2014) 85(8):882–4. doi:10.1136/jnnp-2013-307316
46. Sarasso, E, Agosta, F, Piramide, N, Bianchi, F, Butera, C, Gatti, R, et al. Sensory trick phenomenon in cervical dystonia: A functional MRI study. J Neurol (2020) 267(4):1103–15. doi:10.1007/s00415-019-09683-5
47. Manzo, N, Leodori, G, Ruocco, G, Belvisi, D, Merchant, SHI, Fabbrini, G, et al. Cortical mechanisms of sensory trick in cervical dystonia. Neuroimage Clin (2023) 37:103348. doi:10.1016/j.nicl.2023.103348
48. Medina Escobar, A, Martino, D, and Goodarzi, Z. The prevalence of anxiety in adult-onset isolated dystonia: A systematic review and meta-analysis. Eur J Neurol (2021) 28(12):4238–50. doi:10.1111/ene.15050
49. Sedov, A, Usova, S, Semenova, U, Gamaleya, A, Tomskiy, A, Crawford, JD, et al. The role of pallidum in the neural integrator model of cervical dystonia. Neurobiol Dis (2019) 125:45–54. doi:10.1016/j.nbd.2019.01.011
50. Shaikh, AG, Zee, DS, Crawford, JD, and Jinnah, HA. Cervical dystonia: A neural integrator disorder. Brain (2016) 139(Pt 10):2590–9. doi:10.1093/brain/aww141
51. Szucs, D, and Ioannidis, JP. Sample size evolution in neuroimaging research: An evaluation of highly-cited studies (1990-2012) and of latest practices (2017-2018) in high-impact journals. NeuroImage (2020) 221:117164. doi:10.1016/j.neuroimage.2020.117164
52. Jinnah, HA, and Hess, EJ. Evolving concepts in the pathogenesis of dystonia. Parkinsonism Relat Disord (2018) 46(Suppl. 1):S62–s65. doi:10.1016/j.parkreldis.2017.08.001
53. Trattnig, S, Springer, E, Bogner, W, Hangel, G, Strasser, B, Dymerska, B, et al. Key clinical benefits of neuroimaging at 7T. NeuroImage (2018) 168:477–89. doi:10.1016/j.neuroimage.2016.11.031
54. Burkett, BJ, Fagan, AJ, Felmlee, JP, Black, DF, Lane, JI, Port, JD, et al. Clinical 7-T MRI for neuroradiology: Strengths, weaknesses, and ongoing challenges. Neuroradiology (2021) 63(2):167–77. doi:10.1007/s00234-020-02629-z
55. Valeriani, D, and Simonyan, K. A microstructural neural network biomarker for dystonia diagnosis identified by a DystoniaNet deep learning platform. Proc Natl Acad Sci U S A (2020) 117(42):26398–405. doi:10.1073/pnas.2009165117
56. Alongi, P, Iaccarino, L, and Perani, D. PET neuroimaging: Insights on dystonia and tourette syndrome and potential applications. Front Neurol (2014) 5:183. doi:10.3389/fneur.2014.00183
57. Meringolo, M, Vanni, V, Sciamanna, G, Ponterio, G, Imbriani, P, and Bonsi, P. Vesicular acetylcholine transporter alters cholinergic tone and synaptic plasticity in DYT1 dystonia. Mov Disord (2021) 36:2768–79. doi:10.1002/mds.28698
58. Simonyan, K. Neuroimaging applications in dystonia. Int Rev Neurobiol (2018) 143:1–30. doi:10.1016/bs.irn.2018.09.007
Keywords: dystonia, cervical dystonia, botulinum toxin, magnetoencephalography, neuroimaging, basal ganglia, cerebellum
Citation: Mahajan A (2023) Deconstructing motor and non-motor aspects of dystonia with neuroimaging. Dystonia 2:11526. doi: 10.3389/dyst.2023.11526
Received: 28 April 2023; Accepted: 30 June 2023;
Published: 13 July 2023.
Edited by:
Aasef Shaikh, Case Western Reserve University, United StatesCopyright © 2023 Mahajan. This is an open-access article distributed under the terms of the Creative Commons Attribution License (CC BY). The use, distribution or reproduction in other forums is permitted, provided the original author(s) and the copyright owner(s) are credited and that the original publication in this journal is cited, in accordance with accepted academic practice. No use, distribution or reproduction is permitted which does not comply with these terms.
*Correspondence: Abhimanyu Mahajan, abhimanyumahajan@outlook.com